Editorial
Rev Diabet Stud,
2006,
3(4):156-160 |
DOI 10.1900/RDS.2006.3.156 |
"Persistence of Diabetes" - Why Has Research into Type 1 Diabetes not Made Significant Advances?
Charles Sia1, 2
1Vaccine Center, National Health Research Institutes, 35 Keyan Road, Zhunan Township, Miaoli County, Taiwan.
2Society for Biomedical Diabetes Research, 47057 Duisburg, Germany.
Address correspondence to: Charles Sia, e-mail: siady@nhri.org.tw
Introduction
Diabetes mellitus (DM) constitutes a heterogeneous group of diseases. The two major forms of the disorder can be classified according to insulin dependence, or independence. Insulin-dependent DM, also known as type 1 (T1DM), is a chronic condition manifested by the lack of functional pancreatic islet β-cells which results in failure to produce natural insulin within the body. Without the control mechanism provided by insulin, the body’s blood glucose level cannot be maintained within a physiologically acceptable range. Insulin-independent DM, or type 2 DM (T2DM), is also a chronic condition. It is caused either by insufficient production of insulin by the pancreatic islets, or by the failure of body tissues to process the hormone.
T1DM is the more acute form of the condition and affects approximately 10.0% of all diabetic cases worldwide. As noted above, it is manifested by the lack of insulin-secreting β-cells as a result of their destruction by diabetogenic T cells. T2DM is different in origin, but is also strongly associated with obesity. Despite the different causes of the two forms of diabetes, the outcome is largely the same in both, namely the emergence of hyperglycemia and the potential to cause metabolic disturbances which damage other body systems including nerves and blood vessels.
According to the World Health Organization, the total number of people with T1DM and T2DM was estimated at approximately 30 million in 1985. By 2000, diabetes had increased to an estimated 171 million affected persons [1, 2]. This dramatic increase clearly shows that, in the absence of an effective cure, diabetes is a major uncontrolled and increasing health problem with many of the affected patients continuing to suffer from its complications and an increased risk of premature death. The condition is an enormous burden for patients and economies across the world. DM impairs the quality of life and shortens life-expectancy. As much as 15.0% of the total health care cost in industrialized countries is needed to manage diabetes and to minimize the development of its complications. Despite centuries of seeking an ultimate cure rather than a lifelong treatment that just mitigates the symptoms, no such cure is yet available. So, what are the reasons for the failure of research into diabetes, and what actually needs to be found for a curative therapy? These questions must be addressed if we are to make real progress and set the future direction of diabetes research. This article seeks to revisit the reasons for past failures and to identify future challenges on the avenue of research towards a cure of T1DM, the more acute form of the disease.
Treatment regimens tested in human subjects with T1DM
T2DM and T1DM are treated differently. In the former case, patients are first recommended to commit themselves to changing their lifestyles by adjusting their diet according to nutritional guidelines and doing more exercise. In the majority of cases, these adjustments are not sufficient to control blood glucose levels and patients are put on insulin therapy and glucose-lowering agents (metformin, sulphonylureas). In the case of T1DM on the other hand, the only effective treatment today for T1DM is insulin therapy. This therapy has been available since the discovery made by Banting and Best that insulin extracted from animal pancreatic islets is effective in normalizing blood glucose levels in diabetics [3]. Despite the advancement of this therapy by the development of less reactogenic and immunogenic recombinant human insulin and its analogues, insulin therapy is unable to reverse T1DM. A cure for the disease requires the restoration of functional pancreatic β-cells for the secretion of insulin and the abrogation of autoimmunity.
Approaches designed to replace the diseased pancreas and damaged islet β-cells have involved allogeneic pancreas transplantation and the more recently described islet transplantation. Although much improvement has been made in islet transplantation, as described by Shapiro and colleagues [4], the modified immunosuppressive therapy needed to retain the donor tissues still proves to be toxic for patients. Also, the lack of reproducibility of new stem cell differentiation protocols, together with the limited supply of functional islets, has shown islet transplantation to be an infeasible approach towards a general cure of T1DM.
Following closer observations, the pathogenesis of T1DM has been identified as a T cell-mediated autoimmune disease characterized by the infiltration of autoreactive T cells into the pancreatic islets [5, 6]. Various immunotherapeutic regimens have since been developed to target autoreactive T cells in attempts to prevent or reverse T1DM. Unfortunately, the first line of effort, which is devoted to inhibiting their activity through the use of immunosuppressive drugs and/or anti-lymphocyte antiserum, has met with unacceptable toxicity from these reagents. Clearly, this outweighs their clinical benefits. Further evidence has revealed that circulatory T cells, reactive against islet antigens, are present in diabetic subjects. The next wave of strategies tested to halt T1DM progression involved antigen-specific immunotherapy to tolerize and modify the activity of these autoreactive T cells. However, despite encouraging findings from a pilot study that insulin-reactive T cells can be made tolerant via insulin administration [7], the results could not be confirmed in the large clinical Diabetes Prevention Trial-1 (DPT-1) [8]. Whatever the full interpretation of the DPT-1 results may be, they would be associated with the use of whole insulin as an effective tolerogen.
Alternatively, and perhaps additionally, tolerizing insulin-specific autoreactive T cells could be insufficient to prevent T1DM. Immunotherapies with other forms of islet antigens are ongoing at the present time. Promising results have been gathered from small trials involving the use of an insulin epitope (B9-23) analog [9], recombinant GAD65 protein formed in alum [10], and a modified heat shock protein (HSP) epitope-containing peptide (Diapep277) [11]. However, in recent clinical trials with Diapep277, this reagent did not show promising results in relation to efficacy, as it does not significantly lower HbA1c or daily insulin requirements [12, 13]. Besides antigen-specific immunotherapy, the prevention of T1DM has also been tested via administration of an appropriate dose of a deimmunized form of a monoclonal antibody against CD3 of the human T cell receptor complex. While improvement in insulin secretion has been reported for some patients with TIDM in a small number of diabetics tested [14, 15], the effectiveness of anti-CD3 therapy requires it to be approved for a large trial [16]. The overall safety and efficacy of these immunotherapeutic trials are not yet fully known. Data from large clinical trials are needed for more complete evaluation. At present, it appears that only a combination of low-dose anti-CD3 together with antigen-driven tolerization could be sufficiently effective and safe.
Novel immunotherapeutic developments in the preclinical stage
Over recent years, other means of manipulating diabetogenic T cells and regulatory T cells in the immune system have also been studied. It was found that CD4+ cells from human T1DM patients secrete reduced Th2 cytokine patterns (IL-4 and IL-10) compared to non-diabetics [17]. Attempts to normalize IL-4 and IL-10 levels as preventative treatment for T1DM have been tested in NOD mice and BB rats. The delivery of these cytokines through expression vectors [18, 19] or biodegradable carriers [20] as well as dendritic cells [21] has proved promising, but clinical trials are still outstanding.
Another finding was that NOD mice have a reduced number of natural killer T (NKT) cells in their organs and this has been associated with a higher risk of developing autoimmune diabetes [22]. It has been suggested that a protective mechanism may involve stimulated NKT cells secreting enhanced level of IL-4 which shifts the Th1 phenotype of autoreactive T cells towards their non-diabetogenic Th2 phenotype [23]. There have been attempts to protect diabetic mice from disease onset by the stimulation of lymphocytes through the administration of α-galactosylceramide, an artificial ligand recognized by the CD1 receptor of NKT cells [24, 25]. Despite promising findings with this treatment, there are two unresolved issues that have challenged the application of NKT cell therapy for intervention in T1DM. The first is whether all diabetics have abnormalities in NKT cell numbers and/or functions [26, 27]. The second relates to the high toxicity of α-galactosylceramide reported in mouse studies [28, 29]. Therefore, the potential of NKT cell therapy in T1DM is questionable and further studies are needed to evaluate its safety.
What is needed to cure T1DM?
The tested treatment categories are based on antigen-specific as well as non-specific tolerization mechanisms to control autoreactive T cells. Given the current understanding that T1DM is a complex disease associated with both environmental and genetic factors [30, 31], current attempts to prevent or cure the disease do not include these factors as part of the treatment. Considering that T1DM involves the T cell-mediated destruction of pancreatic β-cells, it seems reasonable that the prevention and remission of the disease must rely on processes which render β-cells functional again. This would need to be accompanied by simultaneous action to halt the ongoing autoimmune process, so that the restored functional β-cells are protected from recurring T cell attacks.
Autoimmunity is attributed to abnormal negative selection of islet antigen-specific T cells in the thymus and the failure to silence these autoreactive lymphocytes in the periphery. In central tolerance, normal thymic expression of islet cell antigens is implicated in inducing apoptotic death of T cells reactive to islet antigens [32-34]. Decreased thymic islet antigen expression has been suggested to contribute to the emergence of autoreactive T cells that escape negative selection in central tolerance [32, 33]. Recently, the expression of self-antigens in thymic medullary epithelial cells has been shown to be regulated by the autoimmune regulator (Aire) gene [35]. These new findings may open up options for controlling islet antigen expression in the thymus. However, as thymic functions are rolled back in adults because of the increasing importance of peripheral immunity, it remains questionable whether approaches that regulate thymic immunity are sufficient and appropriate for a successful intervention in T1DM. Given that procedures to restore the islet antigen level in the thymus are not yet available, immunotherapy to maintain peripheral tolerance appears to be the only option for halting the ongoing immune process causing T1DM. At present, there are no integrated immunotherapeutic approaches to curing the disease. Testing new reagents may yield new insight into the pathological mechanisms and this in turn may lead us to a more refined strategy in the future.
Despite the fact that pancreatic regeneration is known in mammals [36, 37], it remains questionable whether the pancreas can regenerate itself in diabetics. Recently, there has been success in the isolation and characterization of murine pancreatic progenitor cells to induce them to differentiate into mature insulin-secreting islet β-cells [38]. But it is important to confirm that there are human counterparts to these cells and to establich that they are also present in the diseased pancreas. Subsequent understanding of the pathways in the development of these progenitors into functional β-cells, and the development of reagents to induce their differentiation in vivo will be necessary to apply this aspect of the technology as a part of the treatment for T1DM.
Even though integrated and curative therapies are not available today, continuing research in the field of immune tolerance in combination with regenerative strategies is paving the way for novel treatment regimens to cure T1DM. This trend of novel treatment strategies is set to continue in the quest to end the disease. Regrettably, at the moment, no-one can propose a timescale for how long this might take. SBDR members are playing a key part in this by taking a lead, sharing new knowledge and giving more focus to research into diabetes. The spirit of cooperation represents a commitment to move research forward to an eventual cure, rather than settle for palliative treatments. The cover design for this quarter's publication portrays the sense of passing time and frustrations that we all may feel when progress does not seem to be quick enough. Nevertheless, we strongly believe that we can achieve a better future, and that the cover image may yet remain a surreal one (Figure 1).
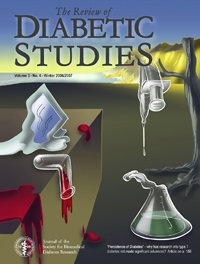 |
 |
Figure 1. Persistence of Diabetes. The cover image is based on Salvador Dali’s surreal painting "Persistence of Memory", 1931. Diabetes also has shown itself to be persistent as centuries of research have not yet found a cure for this disease. Many questions still remain unanswered but we are of good hope that progress in coming research will enable a better future and that the cover image of this issue of The Review of Diabetic Studies will remain a surreal one. |
|
References
- World Health Organization. Diabetes mellitus: report of a WHO study group. Technical Report Series no. 727, WHO, Geneva. [DOD]
- Wild S, Roglic G, Green A, Sicree R, King H. Global prevalence of diabetes: estimates for the year 2000 and projections for 2030. Diabetes Care 2004. 27(5):1047-1053. [DOD]
- Banting FG, Best CH. Pancreatic extracts. J Lab Clin Med 1922. 7:251-266. [DOD]
- Ryan EA, Lakey JR, Paty BW, Imes S, Korbutt GS, Kneteman NM, Bigam D, Rajotte RV, Shapiro AM. Successful islet transplantation: continued insulin reserve provides long-term glycemic control. Diabetes 2002. 51:2148-2157. [DOD]
- Hanninen A, Jalkanen S, Salmi M, Toikkanen S, Nikolakaros G, Simell O. Macrophages, T cell receptor usage, and endothelial cell activation in the pancreas at the onset of insulin-dependent diabetes mellitus. J Clin Invest 1992. 90:1901-1910. [DOD]
- Imagawa A, Hanafusa T, Tamura S, Moriwaki M, Itoh N, Yamamoto K, Iwahashi H, Yamagata K, Waguri M, Nanmo T, et al. Pancreatic biopsy as a procedure for detecting in situ autoimmune phenomenon in type 1 diabetes. Diabetes 2001. 50:1269-1273. [DOD]
- Fuchtenbusch M, Rabl W, Grassl B, Bachmann W, Standl E, Ziegler AG. Delay of type 1 diabetes in high risk, first degree relatives by parental antigen administration: The Schwabing Insulin Prophylaxis Pilot Trial. Diabetologia 1998. 41:536-541. [DOD] [CrossRef]
- Type 1 Diabetes Study Group. Effects of insulin in relatives of patients with type 1 diabetes mellitus. Diabetes Prevention Trial. N Engl J Med 2002. 346:1685-1691. [DOD] [CrossRef]
- Alleva DG, Crowe PD, Jin L, Kwok WW, Ling N, Gottschalk M, Conlon PJ, Gottlieb PA, Putnam AL, Gaur A. A disease-associated cellular immune response in type 1 diabetics to an immunodominant epitope of insulin. J Clin Invest 2001. 107(2):173-180. [DOD]
- Agardh CD, Cilio CM, Lethagen A, Lynch K, Leslie RD, Palmer M, Harris RA, Robertson JA, Lernmark A. Clinical evidence for the safety of GAD65 immunomodulation in adult-onset autoimmune diabetes. J Diabetes Complications 2005. 19(4):238-246. [DOD] [CrossRef]
- Raz I, Elias D, Avron A, Tamir M, Metzger M, Cohen IR. Beta-cell function in new-onset type 1 diabetes and immunomodulation with a heat-shock protein peptide (DiaPep277): a randomised, double-blind, phase II trial. Lancet 2001. 358(9295):1749-1753. [DOD] [CrossRef]
- Schloot NC, Meierhoff G, Lengyel C, Vandorfi G, Takacs J, Panczel P, Barkai L, Madacsy L, Oroszlan T, Kovacs P, Suto G, Battelino T, Hosszufalusi N, Jermendy G. Effect of heat shock protein peptide DiaPep277 on ss-cell function in paediatric and adult patients with recent-onset diabetes mellitus type 1: two prospective, randomized, double-blind phase II trials. Diabetes Metab Res Rev 2007. In press. [DOD]
- Huurman VA, Decochez K, Mathieu C, Cohen IR, Roep BO. Therapy with the hsp60 peptide DiaPep277(trade mark) in C-peptide positive type 1 diabetes patients. Diabetes Metab Res Rev 2007. In press. [DOD]
- Herold KC, Bluestone JA, Montag AG, Parihar A, Wiegner A, Gress RE, Hirsch R. Prevention of autoimmune diabetes with nonactivating anti-CD3 monoclonal antibody. Diabetes 1992. 41:385-391. [DOD]
- Chatenoud L, Bach JF. Questioning four preconceived ideas on immunotherapy of clinical type 1 diabetes: lessons from recent CD3 antibody trials. Rev Diabet Stud 2005. 2(3):116-120. [DOD] [CrossRef]
- Bresson D, von Herrath M. Limitations in immunotherapy with CD3 antibodies: comment on the article by Drs. Chatenoud and Bach. Rev Diabet Stud 2005. 2(4):187-189. [DOD] [CrossRef]
- Rapoport MJ, Mor A, Vardi P, Ramot Y, Winker R, Hindi A, Bistritzer T. Decreased secretion of Th2 cytokines precedes up-regulated and delayed secretion of Th1 cytokines in activated peripheral blood mononuclear cells from patients with insulin-dependent diabetes mellitus. J Autoimmun 1998. 11(6):635-642. [DOD] [CrossRef]
- Ko KS, Lee M, Koh JJ, Kim SW. Combined administration of plasmids encoding IL-4 and IL-10 prevents the development of autoimmune diabetes in nonobese diabetic mice. Mol Ther 2001. 4(4):313-316. [DOD] [CrossRef]
- Goudy K, Song S, Wasserfall C, Zhang YC, Kapturczak M, Muir A, Powers M, Scott-Jorgensen M, Campbell-Thompson M, Crawford JM, Ellis TM, Flotte TR, Atkinson MA. Adeno-associated virus vector-mediated IL-10 gene delivery prevents type 1 diabetes in NOD mice. Proc Natl Acad Sci USA 2001. 98:13913-13918. [DOD] [CrossRef]
- Lee M, Koh JJ, Han SO, Ko KS. Prevention of autoimmune insulitis by delivery of interleukin-4 plasmid using a soluble and biodegradable polymeric carrier. Pharm Res 2002. 19(3):246-249. [DOD] [CrossRef]
- Feili-Hariri M, Falkner DH, Gambotto A, Papworth GD, Watkins SC, Robbins PD, Morel PA. Dendritic cells transduced to express interleukin-4 prevent diabetes in nonobese diabetic mice with advanced insulitis. Hum Gene Ther 2003. 14(1):13-23. [DOD] [CrossRef]
- Poulton LD, Smyth MJ, Hawke CG, Silveira P, Shepherd D, Naidenko OV, Godfrey DI, Baxter AG. Cytometric and functional analyses of NK and NKT cell deficiencies in NOD mice. Int Immunol 2001. 13(7): 887-896. [DOD] [CrossRef]
- Hammond KJ, Poulton LD, Palmisano LJ, Silveira PA, Godfrey DI, Baxter AG. Alpha/beta-T cell receptor (TCR)+CD4-CD8- (NKT) thymocytes prevent insulin-dependent diabetes mellitus in nonobese diabetic (NOD)/Lt mice by the influence of interleukin (IL)-4 and/or IL-10. J Exp Med 1998. 187(7): 1047-1056. [DOD] [CrossRef]
- Hong S, Wilson MT, Serizawa I, Wu L, Singh N, Naidenko OV, Miura T, Haba T, Scherer DC, Wei J, Kronenberg M, Koezuka Y, Van Kaer L. The natural killer T-cell ligand alpha-galactosylceramide prevents autoimmune. Nat Med 2001. 7(9):1052-1056. [DOD] [CrossRef]
- Sharif S, Arreaza GA, Zucker P, Mi QS, Sondhi J, Naidenko OV, Kronenberg M, Koezuka Y, Delovitch TL, Gombert JM, et al. Activation of natural killer T cells by alpha-galactosylceramide treatment prevents. the onset and recurrence of autoimmune type 1 diabetes. Nat Med 2001. 7:1057-1062. [DOD] [CrossRef]
- Kukreja A, Cost G, Marker J, Zhang C, Sun Z, Lin-Su K, Ten S, Sanz M, Exley M, Wilson B, Porcelli S, Maclaren N. Multiple immuno-regulatory defects in type-1 diabetes. J Clin Invest 2002. 109(1):131-140. [DOD] [CrossRef]
- Lee PT, Putnam A, Benlagha K, Teyton L, Gottlieb PA, Bendelac A. Testing the NKT cell hypothesis of human IDDM pathogenesis. J Clin Invest 2002. 110(6):793-800. [DOD] [CrossRef]
- Osman Y, Kawamura T, Naito T, Takeda K, Van Kaer L, Okumura K, Abo T. Activation of hepatic NKT cells and subsequent liver injury following administration of alpha-galactosylceramide. Eur J Immunol 2000. 30(7):1919-1928. [DOD] [CrossRef]
- Major AS, Singh RR, Joyce S, Van Kaer L. The role of invariant natural killer T cells in lupus and atherogenesis. Immunol Res 2006. 34(1):49-66. [DOD] [CrossRef]
- Maclaren N, Atkinson M. Is insulin-dependent diabetes mellitus environmentally induced. N Engl J Med 1992. 327:348-349. [DOD]
- Davies JL, Kawaguchi Y, Bennett ST, Copeman JB, Cordell HJ, Pritchard LE, Reed PW, Gough SC, Jenkins SC, Palmer SM, et al. A genome-wide search for human type 1 diabetes susceptibility genes. Nature 2002. 371(8):130-136. [DOD]
- Sospedra M, Ferrer-Francesch X, Dominguez O, Juan M, Foz-Sala M, Pujol-Borrell R. Transcription of broad range of self-antigens in human thymus suggests a role for central mechanisms in tolerance toward peripheral antigens. J Immunol 1998. 161:5918-5929. [DOD]
- Pugliese A, Zeller M, Fernandez A Jr, Zalcberg LJ, Bartlett RJ, Ricordi C, Pietropaolo M, Eisenbarth GS, Bennett ST, Patel DD. The insulin gene is transcribed in the human thymus and transcription levels correlated with allelic variation at the INS VNTR-IDDM2 susceptibility locus for type 1 diabetes. Nat Genet 1997. 15:293-297. [DOD] [CrossRef]
- Vafiadis P, Bennett ST, Todd JA, Nadeau J, Grabs R, Goodyer CG, Wickramasinghe S, Colle E, Polychronakos C. Insulin expression in human thymus is modulated by INS VNTR alleles at the IDDM2 locus. Nat Genet 1997. 15:289-292. [DOD] [CrossRef]
- DeVoss J, Hou Y, Johannes K, Lu W, Liou GI, Rinn J, Chang H, Caspi R, Fong L, Anderson MS. Spontaneous autoimmunity prevented by thymic expression of a single self-antigen. J Exp Med 2006. 203(12):2727-2735. [DOD] [CrossRef]
- Xu G, Stoffers DA, Habener JF, Bonner-Weir S. Exendin-4 stimulates both beta-cell replication and neogenesis, resulting in increased beta-cell mass and improved glucose tolerance in diabetic rats. Diabetes 1999. 48(12):2270-2276. [DOD]
- Li L, Yi Z, Seno M, Kojima I. Activin A and betacellulin: effect on regeneration of pancreatic beta-cells in neonatal streptozotocin-treated rats. Diabetes 2004. 53(3):608-615. [DOD]
- Suzuki A, Nakauchi H, Taniguchi H. Prospective isolation of multipotent pancreatic progenitors using flow-cytometric cell sorting. Diabetes 2004. 53:2143-2152. [DOD]
|