Editorial
Rev Diabet Stud,
2007,
4(2):68-76 |
DOI 10.1900/RDS.2007.4.68 |
The Cross-Regulatory Relationship Between Human Dendritic and Regulatory T Cells and its Role in Type 1 Diabetes Mellitus
Hsuen-Wen Chang, Yen-Hung Chow, Pele Chong, Charles Sia
Vaccine Center, National Health Research Institutes, Zhunan Township, Miaoli County, Taiwan 350.
Address correspondence to: Charles Sia, e-mail: siady@nhri.org.tw
Keywords: type 1 diabetes, Treg cells, dendritic cells, anergy, antigen
Abstract
Dendritic cells (DCs) and T regulatory (Treg) cells play a crucial role in maintaining the tolerance needed to prevent the onset of autoimmunity that leads to the development of type 1 diabetes mellitus (T1DM). Various experimental studies have shown that human DC subsets are involved in the induction of anergy in T cells and in the differentiation of conventional CD4+ and CD8+ lymphocytes into the respective subtypes of Treg cells. Treg cells, in turn, have been shown to modulate the function of DCs to exhibit tolerogenic properties. To evaluate whether T1DM development is related to abnormalities in DCs and Treg cells, many attempts have been made to characterize these cell types in diabetic individuals and in subjects at risk of developing the disease. This review aims to supply an update on the progress made in these aspects of T1DM research.
Introduction
Type 1 diabetes mellitus (T1DM) development is attributed to the destruction of pancreatic islet β-cells by autoreactive T cells comprising both CD4+ and CD8+ lymphocyte subsets. The generation of autoreactive T cells contributes to the impairment of central and peripheral tolerance which is regulated by subsets of dendritic cells (DCs) and T regulatory (Treg) cells.
In non-diabetic subjects, central tolerance is maintained through negative selection. Thymic T cells expressing high affinity TCRαβ specific for islet antigens are deleted through their engagement with DCs, which display multiple surface-bound self-antigenic peptides in association with major histocompatibility complex (MHC) molecules [1]. A decreased expression level of islet antigens in the thymus may result in suboptimal intra-thymic presentation of DCs and in impaired negative selection of islet antigen-specific T cells. These T cells may then escape into the periphery [2, 3]. Evidence for this cascade of events emerged from the finding that islet antigen-specific CD4+ T cells have been detected in the periphery of at-risk individuals and diabetic subjects [4, 5].
The activity of autoreactive T cells is also controlled by a second security mechanism, namely peripheral tolerance. This mechanism involves the deletion of autoreactive T cells by activation-induced cell death (AICD), anergy induction and suppression by Treg cells. AICD also operates in negative selection in the thymus and plays an important role in building up and shaping the memory pool of lymphocytes after antigen exposure. However, evidence suggesting that abnormal AICD contributes to T1DM development is lacking at the present time [6].
Anergy induction is tightly regulated by DCs [7]. Under various experimental conditions, these cells have also been demonstrated to mediate the generation of Treg cells. In the context of immunoregulation, much interest has been focused on the characterization of DCs and Treg lymphocytes to gain insight into the question of how these cell types mediate their cross-regulatory potential and how this mechanism can be exploited to prevent and possibly reverse T1DM.
Human dendritic cells
Human DCs are distributed in tissues and blood. They are composed of a heterogeneous population of hemapoietic cells which are phenotypically and functionally different [8, 9]. As yet, three main pools of human DCs have been described. The first is the pool of CD1a+ Langerhans cells which are distributed in the epidermis and on mucosal surfaces. The two other main subsets of DCs circulate as immature precursors in the blood and are distributed throughout the body in the tissue interstitium and lymph nodes where they develop into mature DC. These are DC1 or CD11c+ myeloid DCs (mDCs) and DC2 or CD11c- plasmacytoid DCs (pDCs), which are derived from myeloid and lymphoid precursors respectively. Continuing characterization has assigned phenotypes to these lymphoid cells. mDCs can be identified by their expression of a set of "myeloid markers", which include CD11c (a β2 integrin), CD13, CD33 (a member of the immunoglobulin superfamily called immunoglobulin-like transcript 1), CD123L (low level of IL-3Rα-chain) and toll-like receptor 4 (TLR4) that binds bacterial lipopolysaccharide [10, 11]. The relative expression level of CD33 has been used to categorize mDCs into mature (CD33H) and immature (CD33L) subpopulations [12]. mDCs (DC1) reside in peripheral tissues where they are positioned to uptake apoptotic cells and microbial components in the case of pathogen invasion into that tissue. A small fraction of mDCs continuously migrate to the regional lymph nodes to encounter T cells there, and in the absence of preceding contact with microbial components or inflammatory mediators, they remain in an immature state. The presentation of self-antigenic peptides by immature mDCs leads to the differentiation of T cells into Treg instead of effector lymphocytes [13].
Plasmacytoid DCs (DC2) are found in the blood and T cell areas of lymphoid organs. The majority of the antigens they express are not found in mDCs. pDCs are characterized by their poor phagocytic capability and their high expression of CD123 (CD123H) for IL-3 binding and signaling, which is required for their survival and differentiation [14]. In addition, pDCs bear CD4, CD62 ligand (CD62L), CD45RA, ILT3 (immunoglobulin-like transcript 3), as well as blood dendritic cell antigen-2 (BDCA-2) and BDCA-4 antigens [15, 16]. In their immature state, pDC are effectice producers of type 1 interferons (IFN-α) in response to viral stimuli. Unlike mDCs, pDCs have been reported to exhibit greater flexibility in immune-regulation, which either leads to the induction of immunity against foreign antigens or to peripheral tolerance. TLR7 and TLR9 expressed by pDCs facilitate the recognition of foreign antigens, such as single-stranded viral RNA and CpG DNA respectively. TNF-α and TNF-β are the principle cytokines secreted by pDCs, in contrast to mDCs, which produce IL-12 [17-19]. An additional subpopulation of CD11c+ blood DCs, which does not express the CD123 but the BDCA-3 antigen, has also been reported [16].
Association of human DCs with the induction of T cell anergy and Treg cell production
DCs are professional antigen-presenting cells (APCs) that express markedly higher levels of MHC/peptide complex and CD80/86 costimulatory molecules than other APCs such as monocytes/macrophages and B cells. Recent studies have suggested that immature and mature DCs perform differential functions: they deliver either stimulatory or regulatory signals to T cells to drive them towards an effector or regulatory phenotype respectively. While the accessory molecules expressed on DCs and associated with their stimulatory function are well characterized, knowledge on the inhibitory molecules DCs utilize to regulate tolerance is only starting to emerge.
No experimental settings are available at present to study the anergizing and Treg cell induction potential of human DCs in T1DM. Our current understanding regarding how DCs are involved in maintaining peripheral tolerance has come from observations made in other experimental systems. These studies have provided evidence to suggest that DC subsets could mediate anergy induction in T cells and induce the differentiation of naïve and memory T cells into Treg cells.
Induction of anergy in CD4+ and CD8+ lymphocytes has been shown to be mediated by primary (freshly isolated from blood), or cytokine-exposed DCs which retain their immature states. Primary DCs express low levels of MHC class II and CD80/86 molecules. Following uptake of either tetanus toxoid (TT) or topoisomerase 1, they could interact with CD4+ cells and change them to acquire the non-IL-2 secreting (i.e. anergic) phenotype upon exposure to a further cycle of antigen re-stimulation [20]. For experimental settings, immature mDCs could be generated from blood monocytes after culturing them in GM-CSF and IL-4 containing medium [21]. Human DCs derived under these conditions would process thyroid peroxidase (TPO) and present self-antigenic peptides of the protein to TPO-specific clones to render them anergic [22]. Human immature DCs, cultured in the presence of IL-10, show a modest downregulated expression of MHC and CD80/86 molecules, acquire the ability to produce IL-10 and are arrested from undergoing further maturation [23, 24]. These pre-conditioned DCs are found to acquire tolerogenic properties that induce anergy in allospecific or autologous CD4+ cells. They also anergize influenza hemaglutinin-specific CD4+ lymphocytes, as is evident by their significant loss of IL-2- and IFN-γ-producing properties upon re-stimulation with the respective antigens [25]. Apart from rendering CD4+ cells anergic, IL-10-pretreated DCs are also capable of inducing anergy in tyrosinase-specific CD8+ cells, where they are found to be no longer capable of lysing human leukocyte antigen (HLA) class 1-matched targets expressing the corresponding tumor antigen [26].
Primary pDCs are immature and would not readily undergo further differentiation when cultured in different cytokine-conditioned media. However, the function of primary pDCs can be modified by exposing them to various external stimuli. pDCs activated by CD40L after culturing in IL-3 are shown to increase their surface expression of HLA class 1 and class 2 molecules as well as CD54 and CD40 adhesion and CD80/86 costimulatory molecules. These conditioned pDCs secrete IFN-γ and IL-12 and induce polarized differentiation of naïve allogeneic CD4+ cells to exhibit T-helper 1 (Th1) phenotypic characteristics [27]. Interestingly, pDCs activated by CD40L without previous exposure to IL-3 secrete markedly reduced amounts of IL-12 as compared to mDCs treated under the same condition. Naïve allogeneic CD8+ cells co-cultured with CD40L-activated pDCs are rendered anergic, as can be seen in their loss of ability to differentiate to DC2 and lyse B cell blasts isolated from the same donor [28].
DCs are involved in inducing intra- and extra-thymic pools of Treg cells. In the human thymus, CD11c+ DCs are activated by thymic stromal lymphopoietin (TSLP)-expressing thymic epithelial cells (also called Hassall's corpuscles) to express high levels of CD80/86 costimulatory molecules. The activated DCs then acquire the ability to interact with CD4+CD8- T cells in a HLA-restricted manner and drive them to differentiate into nTreg cells bearing the CD4+CD25+FOXP3+ phenotype, which are exported to the periphery [29]. In experimental settings, several studies have reported that immature DCs and in vitro conditioned DCs that have retained their immature status have the capability to drive the differentiation of CD4+ and CD8+ lymphocytes into their respective regulatory phenotypes. CD4+ T cells co-cultured with allogeneic monocyte-derived immature DCs (CD83-) show early expression of cytotoxic T lymphocyte-associated molecule 4 (CTLA-4). These cells would progress and differentiate into Tr1 lymphocytes with IL-10-secreting properties and exhibit unresponsiveness in another cycle of re-stimulation with immature or mature allogeneic DCs isolated from the same donor [30]. Mature DCs, generated in vitro through exposure to TNF-α, anti-CD40 antibody treatment or stimulation with LPS, also exhibit immune-modulatory properties and present antigens to autologous CD4+ lymphocytes, which leads to their conversion into Tr1 cells [31].
The tolerizing property of DCs is typically attributed to the expression of accessory molecules with regulatory properties that mediate counter costimulatory signals to inhibit T cell activation. Thus, GM-CSF- and IL-4 treated human monocytes exposed to the heavy chain of the iron-binding protein, ferritin, a product secreted by melanoma cells, would express high levels of B7-H1. B7-H1 binds to programmed death receptor-1 (PD-1) on T cells to deliver a negative costimulatory signal that drives their differentiation into Tr1 cells [32]. Another phenotypic modification tolerogenic DCs acquire is IL-10 secretion. Immature DCs, derived from GM-CSF- and IL-4-cultured blood monocytes exposed to 1α,25-dihydroxy vitamin D3, produce IL-10 and become less potent in stimulating the proliferation of allogeneic T cells [33].
Whether immature and mature pDCs are capable of inducing the differentiation of Treg cells has been addressed in several studies. Treatment of primary pDC precursors bearing the CD4+CD11c-CD3-CD14-CD1-CD20- phenotype with IL-3 and CD40L results in conditioning of pDCs capable of inducing naïve allogeneic CD8+ cells to differentiate into Tr1 cells that secrete IL-10. These cells effectively suppress the proliferation of autologous naïve CD8+ on allogeneic monocyte stimulation [34]. A continuing study shows that pDCs generated under these conditions are phenotypically mature. They express high levels of ICOSL that interact with ICOS on naïve CD4+ cells to induce their polarized differentiation into IL-10 producing Tr1 cells [35]. Interestingly, apart from mediating polarized Tr1 differentiation of naïve CD4+, pDCs but not CD11c+ DCs, are shown to induce the conversion of memory T lymphocytes into regulatory T cells. This is shown with CD11c+BDCA-4+BDCA-1- pDCs through the presentation of human cytomegalovirus (HCMV) to autologous CD4+CD45RA- T cells isolated from HCMV-infected subjects. The regulatory T cells bear the Tr1-like phenotype because they secrete IL-10, and also IFN-γ, and mediate the suppression of the polyclonal proliferation of autologous T cells to staphylococcal enterotoxin B (SEB) stimulation [36].
Apart from establishing the conditions for DCs to induce the differentiation of Tr1 or TR1-like cells, one study has found that signals generated via CpG ODN (phosphorothioate-modified) binding to TLR9 in lineage- (lin-) CD11c-CD123+ primary pDCs result in upregulated expression of CD40, CD80/86 producing IFN-α, TNF-α, IL-6 but not IL-10, TGF-β, or IL-12. The activated pDCs then acquire the capability to induce the differentiation of naïve allogeneic to a FOXP3-expressing phenotype, CD4+CD25+FOXP3+, characteristic of nTreg cells [37].
Collectively, current available experimental evidence suggests that conventional CD4+ and CD8+ lymphocytes in the periphery could encounter immature DCs, or DCs that are programmed under various conditions and become converted into Tr1 or nTreg cells (Figure 1).
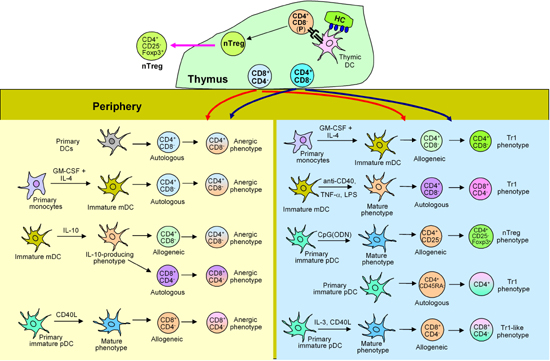 |
 |
Figure 1. DCs in the induction of anergy in CD4+, CD8+ and Treg cells. In the thymus, TSLP-expressing HC (Hassell's corpuscles)-primed DCs interact with a population of CD4+CD8- precursor cells and convert them to nTreg cells. In the periphery, CD4+CD8- and CD8+CD4- lymphocytes, positively selected in the thymus, interact with immature mDCs or pDCs; or these DC subsets programmed under different conditions become anergized or differentiated into Tr1 or nTreg cells. |
|
Modulation of human DC functions by Treg cells
Apart from suppressing effector T cell targets, a few studies have addressed the question of whether the function of DCs is also modulated by Treg cells and of whether this mechanism can contribute to maintaining tolerance. An in vitro study shows that anti-CD3 and IL-2-activated human CD4+CD25+ T cells suppress the maturation of allogeneic DCs generated from GM-CSF- and IL-4-conditioned monocytes. This was verified by the significantly lower level of CD83, HLA-DR and CD86 molecules expressed on CD40L-treated DCs and by the fact that the activated DCs have acquired the property to secrete more IL-10 [38]. Another study shows that anti-CD3- and anti-CD28-activated CD4+CD25high T cells preferentially suppress TLR4-mediated maturation of allogeneic primary CD11c+ DCs and not CpG (ODN) activated BDCA4+ DCs, in a cell-to-cell contact manner by downregulating their surface expression of CD80/86 molecules [39]. Apparently, the regulatory function of CD4+CD25+ T cells activated by anti-CD3 and anti-CD28 monoclonal antibodies could render autologous primary monocytes, primary CD11c+ mDCs as well as GM-CSF- and IL-4 conditioned monocytes to secrete IL-10. This, in turn, acts in an autocrine fashion to trigger the DCs to express B7-H4, a molecule that counteracts the costimulatory signals required for T cell activation [40].
Figure 2 shows how cross-regulation of nTreg cells in the CD4+CD25+ lymphocyte population can induce allogeneic monocytes, CD11c+ mDCs, autologous primary and conditioned monocytes to acquire the tolerogenic IL-10-producing phenotype.
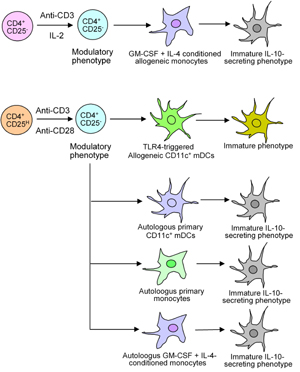 |
 |
Figure 2. Induction of tolerogenic DCs by Treg cells. CD4+CD25+ cells that contain nTreg cells upon activation could halt the maturation of either allogeneic monocyte-derived DCs or primary allogeneic DCs, which exhibit tolerogenic function. Activated CD4+CD25H lymphocytes could mediate the conversion of autologous primary CD11c+ mDCs, autologous primary monocytes, and conditioned monocytes to acquire the IL-10-secreting phenotype with tolerogenic property. |
|
Abnormal DCs in human diabetic individuals
Given that DCs play crucial roles in maintaining peripheral tolerance, which is needed to prevent autoimmunity, investigations have been conducted to address whether abnormalities exist in DC generation and function in diabetic individuals. An early study shows that primary monocytes isolated from individuals between 3 and 58 years of age with established T1DM, who are receiving insulin therapy, exhibit a significant loss of the potential to become differentiated into DCs under in vitro culture conditions. The cells also exhibited a reduced capability to stimulate autologous and allogeneic T cells in the mixed leukocyte reaction (MLR) as compared to those fractionated from non-diabetic controls [41]. Later studies, conducted with monocytes isolated from at-risk children and diabetic adults, have similarly found that freshly isolated monocytes from these groups of subjects possess reduced ability to become differentiated into DCs upon exposure to GM-CSF and IL-4 [42]. Monocytes from recent-onset diabetic children, kept in GM-CSF- and IL-4-conditioned medium, could differentiate normally into DCs [43]. However, results obtained from another study involving a similar cohort of recent-onset T1DM and at-risk children have suggested that monocytes in these individuals are less readily converted into CD11c+ DCs [44]. This shows that the potential of monocytes to differentiate into regulatory phenotypes deteriorates during disease development.
Studies that assess frequency variation and functional changes of primary DCs (not monocyte-derived) in separate cohorts of diabetic subjects have generated mixed results. Staining of blood CD11c+ and CD123+ subsets of DCs in at-risk, recent-onset T1DM and established T1DM subjects reveals that the overall increase in DC numbers in these individuals is significantly higher than in healthy controls. Further analysis of the DC subsets in the diabetic group of individuals shows that a selective reduction in their DC1 cells has occurred and has contributed to a decreased DC1:DC2 ratio as compared to that calculated for non-diabetic subjects. DCs in diabetic subjects exist in a more activated form, based on their higher surface expression of HLA-DR molecules, and exhibit greater IFN-α secreting capability [45]. In adults with established T1DM, the frequencies of their CD11c+ and CD11c- cells are within the range of normal subjects [46]. However, the DCs of these patients exhibit functional defects as they produce a reduced amount of IFN-α following their activation with CD40L [47]. The main observation made from studies conducted in children diagnosed with recent-onset T1DM and young diabetic adults is that their CD11c+ and CD123+ DCs are present at lower frequencies than those detected in age-matched non-diabetic controls. However, DCs from both groups of subjects are found to express comparable level of HLA-DR and CD80/86 molecules and neither of the DC subsets appears to secret abnormal level of cytokines such as IL-2, IL-10, IL-6 and TNF-α [48].
CD11c+ and CD123+ DCs are present in very low numbers as compared to other cell types in the blood [49]. Some investigators have therefore taken the approach that characterizes monocyte-derived DCs in order to evaluate their association with T1DM development. DCs generated from adherent cells in the PBMCs of at-risk children and adults in GM-CSF- and IL-4-supplemented cultures express lower levels of CD80/86, and are significantly less effective in stimulating T cells in autologous and allogeneic MLRs [43]. However, in another similar study performed with recent-onset T1DM and at-risk children, where peripheral blood adherent cells were also used to generate DCs, no aberrant surface expression of CD80 on their DCs was observed [45]. There are two other investigations that have examined DCs generated from CD14+ and CD34+ monocytes cultured in GM-CSF- and IL-4-conditioned medium. However, they report different results from their respective cohorts of diabetic subjects.
CD14+ monocyte-derived DCs from recent-onset T1DM children and age-matched control subjects show similar levels of HLA-DR and CD80/86 molecule expression, cytokine production and stimulatory activity in MLR [44]. Dendritic cells, produced from CD34+ monocytes isolated from children with established T1DM under the same conditions, express lower CD80/86 molecules, secrete less IL-12 but more IL-10 and are less potent in stimulating allogeneic T cells in MLR [50]. Recent studies suggest that DCs generated from CD14+ and CD34+ monocytes are similar in their phenotypic and functional characteristics [51]. It is therefore supposed that the different observations made in these studies are related to the human individuals who were investigated.
Human Treg cells and their association with T1DM
Different subpopulations of Treg cells within the CD4+ and CD8+ subsets regulate the immune responses elicited by effector CD4+ and CD8+ T cells. The best studied cells are the thymus-derived natural Treg (nTreg) cells with the CD4+CD25+FOXP3+ phenotype that could operate through various mechanisms at their disposal in suppressing their CD4+ and CD8+ effector targets [52, 53]. The CD4+ Tr1 cells were generated in vitro from non-activated CD4+ lymphocytes via repeated stimulation with various kinds of antigens in the presence of IL-10. They are functionally characterized as secreting high and moderate levels IL-10 and IFN-γ respectively [54, 55]. Th3 cells are antigen-induced Treg cells that secrete TGF-β as the principle cytokine. They can be generated via oral administration of (auto)antigens. In multiple sclerosis patients they were generated via oral administration of basic protein and proteolipid protein antigen as an immune-intervention treatment [56]. Allospecific CD8+CD28- lymphocytes with suppressive activity against alloreactive CD4+ cells are found in allo- and xenograft transplant subjects [57]. With all these types of Treg cells, attempts have been made to evaluate whether aspects of cell number, frequency, phenotype or function of nTreg are associated with T1DM development.
Frequency analyses of nTreg cells in the PBMCs of human subjects have been carried out in children diagnosed with recent-onset T1DM and in adult diabetics, as well as in the offspring of parents who carry the risk alleles DQA1*05, DQB1*0201 and DQB1*0302. The analyses revealed that the number of circulatory CD4+CD25+ cells was significantly lower in these individuals compared with age-matched controls and individuals whose parents carry low risk alleles (DQB1*0301 and DQB1*0603 and DQB1*0602) [58, 59]. On the other hand, some groups of adults with established T1DM were found to have levels of CD4+CD25+ cells comparable to those detected in HLA-and age-matched non-diabetic individuals [60, 61]. Surprisingly, in a study conducted with a group of LADA patients, the frequencies of CD4+CD25+ cells were found to be even higher than those detected in T2DM and healthy subjects. FOXP3 mRNA analysis revealed that there was significantly less message present in the CD4+ cells of these patients [62]. Evaluation of CD4+CD25high cell frequencies in children diagnosed with recent-onset T1DM and adult diabetics revealed that they are similar to those found in the respective control subjects [63]. For teenagers and young adults diagnosed with recent-onset T1DM and established disease, the frequencies of CD4+CD25+FOXP3+ cells have remained relatively stable over a period of several months and are no different from those detected in age-matched adult healthy controls [61]. The discrepancies in these findings are not clear at the present time. Obviously, T1DM is a complex disease caused by multiple factors. The number of nTreg cells detected in the different groups of patients recruited for the studies may not serve as a primary indicator associated with the stages of the disease which these patients are at.
Information gathered from functional studies suggests that CD4+CD25+ lymphocytes from adult subjects with recent-onset T1DM exhibited reduced capability to suppress mitogen-stimulated proliferation and IFN-γ secretion of autologous CD4+CD25- cells [64]. Dysfunction of CD4+CD25+ is also found in children with recent-onset T1DM and adult diabetics as judged by their significant loss of suppressive activity against anti-CD3 and anti-CD28 stimulated autologous CD4+CD25- cells [64].
In contrast to nTreg lymphocytes, Tr1 cells specific for islet antigens have not been detected in diabetic subjects at the present time. It is however, worth mentioning that glutamic acid decarboxylase (GAD)-specific Tr1 cells with an IL-10 secreting phenotype are found in prediabetic NOD mice. They could be isolated with tetramers containing GAD epitopes. They were observed to suppress cytokine production of CD4+ lymphocytes upon stimulation with GAD peptides and to confer protection in prediabetic animals [65]. Another study demonstrated that Tr1, together with nTreg (CD4+CD25+FoxP3+) cells, can induce tolerance by a combined administration of rapamycin and IL-10 in prediabetic NOD mice. While nTreg cells accumulate in the pancreas, Tr1 lymphocytes are located in the spleen to stop the escape of autoreactive T cells into the draining lymph nodes, thus preventing them from reaching the pancreatic islets of the diabetic animals [66].
Conclusion
Observations made from various in vitro experimental systems have contributed to our understanding of the involvement of DC subsets in the induction of anergy in CD4+ and CD8+ lymphocytes. We have also learned how Treg cells differentiate from conventional CD4+ and CD8+ cells. However, experiments are missing which show if DCs from diabetic subjects could render islet antigen-specific T cells anergic or cause polarized differentiation of conventional T cells to Treg cells. Recent studies have also suggested that T reg cells are able to cross-regulate the function of DCs. How effectively this mechanism works in diabetic subjects needs to be clarified.
The many studies undertaken to characterize the phenotypic and functional characteristics of DC subsets and Treg cells in T1DM individuals are valuable, as they could generate information to answer many questions regarding the relation between DCs and Treg cells in the T1DM setting. Unfortunately, mixed results have been reported from these studies. Continuing efforts are therefore needed to clarify these discrepancies and to provide downstream design of novel immune-intervention strategies for the prevention of T1DM development.
References
- Garcia CA, Prabakar KR, Diez J, Cao ZA, Allende G, Zeller M, Dogra R, Mendez A, Rosenkranz E, Dahl U, Ricordi C, Hanahan D, Pugliese A. Dendritic cells in human thymus and periphery display a proinsulin epitope in a transcription-dependent, capture-independent fashion. J Immunol 2005. 175:2111-2122. [DOD]
- Sospedra M, Ferrer-Francesch X, Dominguez O, Juan M, Foz-Sala M, Pujol-Borrell R. Transcription of broad range of self-antigens in human thymus suggests a role for central mechanisms in tolerance toward peripheral antigens. J Immunol 1998. 161:5918-5929. [DOD]
- Pugliese A, Zeller M, Fernandez A Jr, Zalcberg LJ, Bartlett RJ, Ricordi C, Pietropaolo M, Eisenbarth GS, Bennett ST, Patel DD. The insulin gene is transcribed in the human thymus and transcription levels correlated with allelic variation at the INS VNTR-IDDM2 susceptibility locus for type 1 diabetes. Nat Genet 1997. 15:293-297. [DOD] [CrossRef]
- Mannering SI, Morris JS, Stone NI, Jensen KP, van Endert PM, Harrison LC. CD4+ T cell proliferation in response to GAD and proinsulin in healthy, pre-diabetic, and diabetic donors. Ann NY Acad Sci 2004. 1037:16-21. [DOD] [CrossRef]
- Oling V, Marttila J, Ilonen J, Kwok WW, Nepom G, Knip M, Simell O, Reijonen H. GAD65- and proinsulin-specific CD4+ T-cells detected by MHC class II tetramers in peripheral blood of type 1 diabetes patients and at-risk subjects. J Autoimmun 2005. 25(3):235-243. [DOD] [CrossRef]
- Gronski MA, Weinem M. Death pathways in T cell homeostasis and their role in autoimmune diabetes. Rev Diabet Stud 2006. 3(2):88-95. [DOD] [CrossRef]
- Steinman RM, Hawiger D, Nussenzweig MC. Tolerogenic dendritic cells. Annu Rev Immunol 2003. 21:685-711. [DOD] [CrossRef]
- Steinman RM, Pack M, Inaba K. Dendritic cell development and maturation. In: Ricciardi-Castagnoli P (ed.). Dendritic cells in fundamental and clinical immunology. Vol. 3, Plenum Press, New York, 1997, p. 1-6. [DOD]
- Ardavin C, Martinez del Hoyo G, Martin P, Anjuere F, Arias CF, Marin AR, Ruiz S, Parrillas V, Hernandez H. Origin and differentiation of dendritic cells. Trends Immunol 2001. 22(12):691-700. [DOD] [CrossRef]
- Olweus J, BitMansour A, Warnke R, Thompson PA, Carballido J, Picker LJ, Lund-Johansen F. Dendritic cell ontogeny: a human dendritic cell lineage of myeloid origin. Proc Natl Acad Sci USA 1997. 94(23):12551-12556. [DOD] [CrossRef]
- Coccia EM, Severa M, Giacomini E, Monneron D, Remoli ME, Julkunen I, Cella M, Lande R, Uze G. Viral infection and toll-like receptor agonists induce a differential expression of type I and lambda interferons in human plasmacytoid and monocyte-derived dendritic cells. Eur J Immunol 2004. 34(3):796-805. [DOD] [CrossRef]
- Thomas R, Lipsky PE. Human peripheral blood dendritic cell subsets. Isolation and characterization of precursor and mature antigen-presenting cells. J Immunol 1994. 153(9):4016-4028. [DOD]
- Rissoan MC, Soumelis V, Kadowaki N, Grouard G, Briere F, de Waal Malefyt R, Liu YJ. Reciprocal control of T helper cell and dendritic cell differentiation. Science 1999. 283(5405):1183-1186. [DOD] [CrossRef]
- Kohrgruber N, Halanek N, Groger M, Winter D, Rappersberger K, Schmitt-Egenolf M, Stingl G, Maurer D. Survival, maturation, and function of CD11c- and CD11c+ peripheral blood dendritic cells are differentially regulated by cytokines. J Immunol 1999. 163:3250-3259. [DOD]
- Grouard G, Rissoan MC, Filgueira L, Durand I, Banchereau J, Liu YJ. The enigmatic plasmacytoid T cells develop into dendritic cells with interleukin (IL)-3 and CD40-ligand. J Exp Med 1997. 185(6):1101-1111. [DOD] [CrossRef]
- Dzionek A, Fuchs A, Schmidt P, Cremer S, Zysk M, Miltenyi S, Buck DW, Schmitz J. BDCA-2, BDCA-3 and BDCA-4: three markers for distinct subsets of dendritic cells in human peripheral blood. J Immunol 2000. 165(11):6037-6046. [DOD]
- Ito T, Wang YH, Liu YJ. Plasmacytoid dendritic cell precursors/type I interferon-producing cells sense viral infection by Toll-like receptor (TLR) 7 and TLR9. Springer Semin Immunopathol 2005. 26(3):221-229. [DOD] [CrossRef]
- Cella M, Jarrossay D, Facchetti F, Alebardi O, Nakajima H, Lanzavecchia A, Colonna M. Plasmacytoid monocytes migrate to inflamed lymph nodes and produce large amounts of type I interferon. Nat Med 1999. 5(8):919-923. [DOD] [CrossRef]
- Siegal FP, Kadowaki N, Shodell M, Fitzgerald-Bocarsly PA, Shah K, Ho S, Antonenko S, Liu YJ. The nature of the principal type 1 interferon-producing cells in human blood. Science 1999. 284(5421):1835-1837. [DOD] [CrossRef]
- Kuwana M, Kaburaki J, Wright TM, Kawakami T, Ikeda Y. Induction of antigen-specific human CD4+ T cell anergy by peripheral blood DC2 precursors. Eur J Immunol 2001. 31(9):2547-2557. [DOD] [CrossRef]
- Sallusto F, Lanzavecchia A. Efficient presentation of soluble antigen by cultured human dendritic cells is maintained by granulocyte/macrophage colony-stimulating factor plus interleukin 4 and downregulated by tumor necrosis factor alpha. J Exp Med 1994. 179:1109-1118. [DOD] [CrossRef]
- Quaratino S, Duddy LP, Londei M. Fully competent dendritic cells as inducers of T cell anergy in autoimmunity. Proc Natl Acad Sci USA 2000. 97(20):10911-10916. [DOD] [CrossRef]
- Buelens C, Willems F, Delvaux A, Pierard G, Delville JP, Velu T, Goldman M. Interleukin-10 differentially regulates B7-1 (CD80) and B7-2 (CD86) expression on human peripheral blood dendritic cells. Eur J Immunol 1995. 25:2668-2672. [DOD]
- Corinti S, Albanesi C, la Sala A, Pastore S, Girolomoni G. Regulatory activity of autocrine IL-10 on dendritic cell functions. J Immunol 2001. 166:4312-4318. [DOD]
- Steinbrink K, Wolfl M, Jonuleit H, Knop J, Enk AH. Induction of tolerance by IL-10-treated dendritic cells. J Immunol 1997. 159(10):4772-4780. [DOD]
- Steinbrink K, Jonuleit H, Muller G, Schuler G, Knop J, Enk AH. Interleukin-10-treated human dendritic cells induce a melanoma-antigen-specific anergy in CD8+ T cells resulting in a failure to lyse tumor cells. Blood 1999. 93(5):1634-1642. [DOD]
- Cella M, Facchetti F, Lanzavecchia A, Colonna M. Plasmacytoid dendritic cells activated by influenza virus and CD40L drive a potent Th1 polarization. Nat Immunol 2000. 1:305-310. [DOD] [CrossRef]
- Rissoan MC, Soumelis V, Kadowaki N, Grouard G, Briere F, de Waal Malefyt R, Liu YJ. Reciprocal Control of T Helper Cell and Dendritic Cell Differentiation. Science 1999. 283(5405):1183-2001. [DOD]
- Watanabe N, Wang YH, Lee HK, Ito T, Wang YH, Cao W, Liu YJ. Hassall's corpuscles instruct dendritic cells to induce CD4+CD25+ regulatory T cells in human thymus. Nature 2005. 436:1181-1185. [DOD] [CrossRef]
- Jonuleit H, Schmitt E, Schuler G, Knop J, Enk AH. Induction of interleukin 10-producing, nonproliferating CD4+ T cells with regulatory properties by repetitive stimulation with allogeneic immature human dendritic cells. J Exp Med 2000. 192(9):1213-1222. [DOD] [CrossRef]
- Lundqvist A, Palmborg A, Pavlenko M, Levitskaya J, Pisa P. Mature dendritic cells induce tumor-specific type 1 regulatory T cells. J Immunother (1997) 2005. 28(3):229-235. [DOD] [CrossRef]
- Gray CP, Arosio P, Hersey P. Heavy chain ferritin activates regulatory T cells by induction of changes in dendritic cells. Blood 2002. 99(9):3326-3334. [DOD] [CrossRef]
- Penna G, Adorini L. 1alpha,25-dihydroxyvitamin D3 inhibits differentiation, maturation, activation, and survival of dendritic cells leading to impaired alloreactive T cell activation. J Immunol 2000. 164(5):2405-2411. [DOD]
- Gilliet M, Liu YJ. Generation of human CD8 T regulatory cells by CD40 ligand-activated plasmacytoid dendritic cells. J Exp Med 2002. 195(6):695-704. [DOD] [CrossRef]
- Ito T, Yang M, Wang YH, Lande R, Gregorio J, Perng OA, Qin XF, Liu YJ, Gilliet M. Plasmacytoid dendritic cells prime IL-10-producing T regulatory cells by inducible costimulator ligand. J Exp Med 2007. 204(1):105-115. [DOD] [CrossRef]
- Kvale EO, Floisand Y, Lund-Johansen F, Rollag H, Farkas L, Ghanekar S, Brandtzaeg P, Jahnsen JL, Olweus J. Plasmacytoid DCs regulate recall reponses by rapid induction of IL-10 in memory T cells. Blood 2007. 109(8):3369-3376. [DOD] [CrossRef]
- Moseman A, Liang X, Dawson AJ, Panoskaltsis-Mortari A, Krieg AM, Liu YJ, Blazar BR, Chen W. Human plasmacytoid dendritic cells activated by CpG oligodeoxynucleotides induce the generation of CD4+CD25+ regulatory T cells. J Immunol 2004. 173:4433-4442. [DOD]
- Misra N, Bayry J, Lacroix-Desmazes S, Kazatchkine MD, Kavri SV. Cutting edge: human CD4+CD25+ T cells restrain the maturation and antigen-presenting function of dendritic cells. J Immunol 2004. 172:4676-4680. [DOD]
- Houot R, Perrot I, Garcia E, Durand I, Lebecque S. Human CD4+CD25high regulatory T cells modulate myeloid but not plasmacytoid dendritic cells activation. J Immunol 2006. 176:5293-5298. [DOD]
- Kryczek I, Wei S, Zou L, Zhu G, Mottram P, Xu H, Chen L, Zou W. Cutting edge: induction of B7-H4 on APCs through IL-10: novel suppressive mode for regulatory T cells. J Immunol 2006. 177:40-44. [DOD]
- Jansen A, van Hagen M, Drexhage HA. Defective maturation and function of antigen-presenting cells in type 1 diabetes. Lancet 1995. 345:491-492. [DOD] [CrossRef]
- Takahashi K, Honeyman MC, Harrison LC. Impaired yield, phenotype, and function of monocyte-derived dendritic cells in humans at risk for insulin-dependent diabetes. J Immunol 1998. 161:2629-2635. [DOD]
- Zacher T, Knerr I, Rascher W, Kalden JR, Wassmuth R. Characterization of monocyte-derived dendritic cells in recent-onset diabetes mellitus type 1. Clin Immunol 2002. 105(1):17-24. [DOD] [CrossRef]
- Skarsvik S, Tiittanen M, Lindstrom A, Casas R, Ludvigsson J, Vaarala O. Poor in vitro maturation and pro-inflammatory cytokine response of dendritic cells in children at genetic risk of type 1 diabetes. Scand J Immunol 2004. 60(6):647-652. [DOD] [CrossRef]
- Peng R, Li Y, Brezner K, Litherland S, Clare-Salzler MJ. Abnormal peripheral blood dendritic cell populations in type 1 diabetes. Ann NY Acad Sci 2003. 1005(1):222-225. [DOD] [CrossRef]
- Summers KL, Behme MT, Mahon JL, Singh B. Characterization of dendritic cells in humans with type 1 diabetes. Ann NY Acad Sci 2003. 1005:222-229. [DOD] [CrossRef]
- Summers KL, Marleau AM, Mahon JL, McManus R, Hramiak I, Singh B. Reduced IFN-alpha secretion by blood dendritic cells in human diabetes. Clin Immunol 2006. 121(1):81-89. [DOD] [CrossRef]
- Vuckovic S, Withers G, Harris M, Khalil D, Gardiner D, Flesch I, Tepes S, Greer R, Cowley D, Cotterill A, Hart DN. Decreased blood dendritic cell counts in type 1 diabetic children. Clin Immunol 2007. 123:281-288. [DOD] [CrossRef]
- Szabolcs P, Park KD, Reese M, Marti L, Broadwater G, Kurtzberg J. Absolute values of dendritic cell subsets in bone marrow, cord blood, and peripheral blood enumerated by a novel method. Stem Cells 2003. 21(3):296-303. [DOD] [CrossRef]
- Angelini F, Del Duca E, Piccinini S, Pacciani V, Rossi P, Manca Bitti ML. Altered phenotype and function of dendritic cells in children with type 1 diabetes. Clin Exp Immunol 2005. 142(2):341-346. [DOD] [CrossRef]
- Syme R, Bajwa R, Robertson L, Stewart D, Gluck S. Comparison of CD34 and monocyte-derived dendritic cells from mobilized peripheral blood from cancer patients. Stem Cells 2005. 23(1):74-81. [DOD] [CrossRef]
- Sakaguchi S. Naturally arising Foxp3-expressing CD25+CD4+ regulatory T cells in immunological tolerance to self and non-self. Nat Immunol 2005. 6(4):345-352. [DOD] [CrossRef]
- von Boehmer H. Mechanisms of suppression by suppressor T cells. Nat Immunol 2005. 6:338-344. [DOD] [CrossRef]
- Groux H, O'Garra A, Bigler M, Rouleau M, Antonenko S, de Vries JE, Roncarolo MG. A CD4+ T-cell subset inhibits antigen-specific T-cell responses and prevents colitis. Nature 1997. 389(6652):737-742. [DOD]
- Roncarolo MG, Gregori S, Battaglia M, Bacchetta R, Fleischhauer K, Levings MK. Interleukin-10-secreting type 1 regulatory T cells in rodents and humans. Immunol Rev 2006. 212:28-50. [DOD] [CrossRef]
- Fukaura H, Kent SC, Pietrusewicz MJ, Khoury SJ, Weiner HL, Hafler DA. Induction of circulating myelin basic protein and proteolipid protein-specific transforming growth factor-beta1-secreting Th3 T cells by oral administration of myelin in multiple sclerosis patients. J Clin Invest 1996. 98(1):70-77. [DOD]
- Ciubotariu R, Vasilescu R, Ho E, Cinti P, Cancedda C, Poli L, Late M, Liu Z, Berloco P, Cortesini R, Suciu-Foca Cortesini N. Detection of T suppressor cells in patients with organ allografts. Hum Immunol 2001. 62(1):15-20. [DOD] [CrossRef]
- Kukreja A, Cost G, Marker J, Zhang C, Sun Z, Su KL, Ten S, Sanz M, Exley M, Wilson B, Porcelli S, Maclaren N. Multiple immuno-regulatory defects in type-1 diabetes. J Clin Invest 2002. 109(1):131-140. [DOD] [CrossRef]
- Michalek J, Vrabelova Z, Hrotekova Z, Kyr M, Pejchlova M, Kolouskova S, Faresjo M, Stechova K. Immune regulatory T cells in siblings of children suffering from type 1 diabetes mellitus. Scand J Immunol 2006. 64:531-535. [DOD] [CrossRef]
- Lindley S, Dayan CM, Bishop A, Roep BO, Peakman M, Tree TI. Defective suppressor function in CD4+CD25+ T-cells from patients with type 1 diabetes. Diabetes 2005. 54(1):92-99. [DOD] [CrossRef]
- Putnam AL, Vendrame F, Dotta F, Gottlieb PA. CD4+CD25high regulatory T cells in human autoimmune diabetes. J Autoimmunity 2005. 24(1):55-62. [DOD] [CrossRef]
- Yang Z, Zhou Z, Huang G, Ling H, Yan X, Peng J, Li X. The CD4+ regulatory T-cells is decreased in adults with latent autoimmune diabetes. Diab Res Clin Pract 2007. 76(1):126-131. [DOD] [CrossRef]
- Baecher-Allan C, Brown JA, Freeman GJ, Hafler DA. CD4+CD25high regulatory cells in human peripheral blood. J Immunol 2001. 167(3):1245-1253. [DOD]
- Brusko T, Wasserfall C, McGrail K, Schatz R, Viener HL, Schatz D, Haller M, Rockell J, Gottlieb P, Clare-Salzler M, Atkinson M. No alterations in the frequency of FOXP3+ regulatory T-cells in type 1 diabetes. Diabetes 2007. 56(3):604-612. [DOD] [CrossRef]
- Chen C, Lee WH, Yun P, Snow P, Liu CP. Induction of autoantigen-specific Th2 and Tr1 regulatory T cells and modulation of autoimmune diabetes. J Immunol 2003. 171:733-744. [DOD]
- Battaglia M, Stabilini A, Draghici E, Migliavacca B, Gregori S, Bonifacio E, Roncarolo MG. Induction of tolerance in type 1 diabetes via both CD4+CD25+ T regulatory cells and T regulatory type 1 cells. Diabetes 2006. 55(6):1571-1580. [DOD] [CrossRef]
This article has been cited by other articles:
|
From Toll-like receptors to the toll house of type 1 diabetes mellitus
Luft FC
J Mol Med 2010. 88(12):1191-1194
|
|
|
Functional alterations of proinflammatory monocytes by T regulatory cells: implications for the prevention and reversal of type 1 diabetes
Sia C, Hänninen A
Rev Diabet Stud 2010. 7(1):6-14
|
|
|
Dendritic Cell Transfected with Secondary Lymphoid-Tissue Chemokine and/or Interleukin-2 Gene-Enhanced Cytotoxicity of T-Lymphocyte in Human Bladder Tumor Cell S In Vitro
Li YG, Wang ZP, Tian JQ, Tian BQ, Rodrigues R, Shang PF, Zhang T
Cancer Invest 2009. 27(9):909-917
|
|
|
The significance of Treg cells in defective tumor immunity
Kosmaczewska A, Ciszak L, Potoczek S, Frydecka I
Arch Immunol Ther Exp 2008. 56(3):181-191
|
|
|