Editorial
Rev Diabet Stud,
2008,
5(1):6-12 |
DOI 10.1900/RDS.2008.5.6 |
Imaging the Beta-Cell Mass: Why and How
Frantisek Saudek1, Carl-Henrik Brogren2, Srirang Manohar3
1Diabetes Center, Institute for Clinical and Experimental Medicine, Videnska 1958/9, 14021 Prague 4, Czech Republic
2Department of Biomedical Sciences, University of Copenhagen, Blegdamsvej 3, DK-2200 Copenhagen N, Denmark
3Biophysical Engineering Group, Institute for Biomedical Technology, University of Twente, PB217, 7500AE Enschede, The Netherlands
Address correspondence to: Frantisek Saudek, e-mail: frantisek.saudek@ikem.cz
Manuscript submitted May 21, 2008; accepted May 29, 2008.
Keywords: diabetes, beta-cell, noninvasive imaging, IC2, islet transplantation, photoacoustic, MRI, PET
Abstract
Diabetes is a disorder characterized by beta-cell loss or exhaustion and insulin deficiency. At present, knowledge is lacking on the underlying causes and for the therapeutic recovery of the beta-cell mass. A better understanding of diabetes pathogenesis could be obtained through exact monitoring of the fate of beta-cells under disease and therapy conditions. This could pave the way for a new era of intervention by islet replacement and regeneration regimens. Monitoring the beta-cell mass requires a reliable method for noninvasive in vivo imaging. Such a method is not available at present due to the lack of a beta-cell-specific contrast agent. The only existing method to monitor islet cells in vivo consists of labeling islet transplants with iron nanoparticles prior to transplantation and visualization of the transplanted islets by magnetic resonance imaging (MRI). Therefore, accurate assessment of the native beta-cell mass is still limited to autopsy studies. Endeavors to find a biological structure specific for beta-cells led to the discovery of potential candidates that have been tested for noninvasive imaging. Among them are the ligand to the vesicular monoamine transporter type 2 (VMAT-2), which is called dihydrotetrabenazine (DTBZ), antibodies to zinc transporter (ZnT-8) and the monoclonal antibody IC2. While DTBZ and antibodies to ZnT-8 showed binding activities to more than beta-cells, the anti-IC2 monoclonal antibody showed binding properties exclusively to insulin-producing beta-cells. This effect was demonstrated in many previous investigations, and has been further substantiated more recently. Thus, at present, IC2 seems to be the only useful marker for noninvasive functional imaging of native beta-cells. Experiments with a radioisotope-chelated IC2 structure on pancreas ex vivo showed that the tracer specifically bound to the beta-cell surface and could be detected by nuclear imaging. In the near future, these promising findings may offer a new way to monitor the beta-cell mass in vivo under disease and therapy conditions so that we can learn more about diabetes pathogenesis and options for disease prevention.
Introduction
Diabetes mellitus is a metabolic disorder characterized by high blood glucose levels, which result from an absolute or relative deficiency in insulin secretion. In type 1 diabetes, insulin-producing beta-cells are mainly destroyed by an autoimmune process, whereas in type 2 diabetes they cannot meet the increasing metabolic demands and get exhausted. In addition to regimen intervention, current therapeutic approaches are mostly based on insulin substitution, stimulation of endogenous insulin production and improvement of insulin action. Although both types of diabetes can be - more or less successfully - medically controlled, the disease lasts a lifetime and is accompanied by serious late organ complications.
Despite the fact that the underlying causes of both types of diabetes have not yet been fully discovered, in recent years there has been remarkable progress in understanding their pathogenesis, which has opened up new therapeutic opportunities. It has been shown that the life-span of differentiated beta-cells is limited, and their natural renewal happens both in experimental animals and in humans [1, 2]. The renewal of beta-cells may originate from beta-cell replication or differentiation from putative adult stem cells. Immune-mediated and metabolic injuries lead to functional and later morphological beta-cell impairment. In diabetes, the amount of functional beta-cells decreases during the course of the disease and natural renewal cannot counteract this process [3]. Therefore, the relevant aspect for the occurrence of diabetes is the mass of surviving beta-cells. While insulin sensitivity as an important aspect of glucose tolerance can be quantified by glucose clamp techniques, reliable methods for the assessment of the beta-cell mass are still lacking.
Need for noninvasive beta-cell imaging
The development and implementation of noninvasive techniques for the quantitative measurement of the functional beta-cell mass and the spatial visualization of its component would help in the early diagnosis of beta-cell loss or dysfunction in pre-clinical phases of diabetes. Furthermore, it would enable evaluation of emerging therapeutic approaches which specifically focus on preservation or regeneration of beta-cells. These include, for example, early immune intervention at the level of autoreactive T cells [4, 5] and tolerance induction by expansion of regulatory T cell compartments in type 1 diabetes [6]. For both diabetes types, researchers are endeavoring to reduce the rate of beta-cell apoptosis and stimulate islet growths and neogenesis using GLP-1 [7] or gastrin analogues [8]. Another approach is to block GLP-1 cleavage [9].
Until recently, only indirect methods were available. These relied on measuring insulin secretion following different metabolic stimuli. Direct visualization of either native or transplanted pancreatic islets was unsuccessful due to their small size, little difference in physical characteristics from the surrounding tissue, and their relatively low number dispersed over a large area of the pancreas or the liver as the most common site of transplantation. However, modern diagnostic equipment can provide very high sensitivity (by positron emission tomography (PET) and single photon emission computed tomography (SPECT)), spatial resolution (by magnetic resonance imaging (MRI)) or both (by PET/CT). Despite these advances in imaging devices, the main problem is the lack of a specific structural or molecular marker to enable differentiation between scattered islets or single beta-cells, and surrounding tissue.
Noninvasive imaging of transplanted islets
So far, MRI is the only method to visualize beta-cells successfully in vivo, due to its capability to resolve to 0.1 mm and less, depending on the device. However, this method requires in vitro islet labeling prior to transplantation [10, 11]. During a 8-48 hour incubation period in the presence of non-toxic iron oxide crystals coated by carboxytextrane (particle size 60-100 nm) iron accumulates in all major islet cell types including macrophages (Figure 1). In T2-weighted images, iron shows a strong superparamagnetic effect that enables the visualization of individual islets or their clusters following transplantation as distinct hypointense spots located in the liver parenchyma (Figure 2).
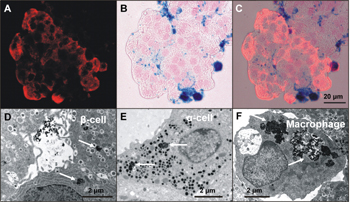 |
 |
Figure 1. Rat islets labeled with ironoxide nanoparticles (ferucarbotran). A: Immunodetection of C-peptide on a frozen section (red). B: Histochemical detection of iron (Fe3+). Prussian blue reaction (blue) on the same section. C: Iron localization in beta-cells in a combined view. D-F: Transmission electron micrographs show iron particles (arrows) inside beta-cells (D), alpha-cell (E) and an islet macrophage (F). |
|
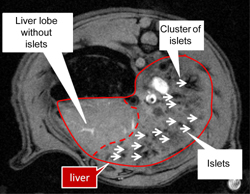 |
 |
Figure 2. T2-weighted MR image of a rat liver in vivo with 2000 transplanted human pancreatic islets labeled by superparamagnetic iron oxide nanoparticles Resovist (r). |
|
In experiment, at least, this way of islet imaging correlates with their survival, or rejection, over a several months period [12]. Functional survival immediately following transplantation can be documented by PET or PET/CT imaging after previous in vitro labeling with 2-[18F]fluoro-2-deoxy-D-glucose [13, 14]. While the spatial resolution of PET is rather low, the combination with CT significantly improves the anatomic correlation.
In the near future, clinical MRI of the islet graft should be possible with the use of a medically approved contrast agent applied for islet labeling in vitro. Preliminary results in humans confirmed the feasibility of the approach and the safety of the contrast agent [15]. In the next step, more effective labeling methods, techniques of automatic quantitative assessment of islet mass, faster protocols with better reduction of breathing motion artifacts and better spatial resolution, will be developed.
According to the Clinical Trial website, a pilot study is currently under way in type 1 diabetes and recipients of pancreatic graft using 11C-Dihydro-tetrabenazine and PET scan will show, whether or not this approach is able to quantify the beta-cell mass in relation to conventional metabolic tests. It is likely that future studies assessing the native beta-cell mass will be based on beta-cell specific antibodies, or humanized high affinity fragments, or ligands, which will be conjugated with either radioactive probes or MR contrast probes. However, the most important remaining challenge is to find the right target structure.
Search for a beta-cell-specific probe for imaging the native beta-cell mass
At present, only nonspecific MR contrast agents or PET probes, based on characteristic biological and physical-chemical properties of different tissues are available in diagnostics. These contrast agents do not allow the discrimination of such small objects as individual pancreatic islets or beta-cells without their previous ex vivo labeling. Obviously, only a new generation of specifically targeted contrast agents engineered by different principles will allow monitoring of the fate of native beta-cells in vivo.
The successful candidate for a new generation of beta-cell-specific contrast agent should meet the following criteria: 1. it should be a marker expressed exclusively by beta-cells and not by any other pancreatic cells; 2. it should be expressed in a sufficient amount to be available for the imaging probe; and 3. tissue surrounding the pancreas should be devoid of this marker to prevent high background signal from these organs during imaging. It is estimated that to be effective, such a structure needs to be present in a concentration 100 times higher than in the surrounding tissue [16].
This can only be achieved by using a beta-cell-targeted structure that is sufficiently specific. Several candidate substances, such as L-DOPA, glibenclamide, tolbutamide, serotonin, nicotinamide, fluorodeoxyglucose, fluorodithisone [16] or islet-specific GAD, IA2 and K14D10 antibodies [17], have been tested. Unfortunately, none of the tested structures sufficiently accumulated in the pancreas or provided satisfactory specificity.
Three novel beta-cell targets have raised interest to date. Among these new candidates is the vesicular monoamine transporter type 2 (VMAT-2). This was found as islet-localized protein using a functional genomic approach [18], and its ligand dihydrotetrabenazine (DTBZ) is currently under consideration as a tracer for imaging techniques in human studies. Although VMAT-2 in the pancreas is expressed in endocrine and not in exocrine tissue, it is a marker of monoaminergic neurons in the brain. It is present in vesicles and expressed by cells of many other tissues than endocrine pancreas, such as endometrial and gastrointestinal tissues [19, 20]. This will cause low specificity of its ligand to beta-cells, which does not make DTBZ a useful structure applicable for noninvasive beta-cell imaging.
Recently, the ZnT-8 protein has been reported as a beta-cell-specific structure [21]. ZnT-8 is an intracellular protein supposedly responsible for cellular efflux of zinc into the extracellular matrix or into intracellular vesicles, zinc accumulation in the insulin granule lumen and provision of zinc for insulin maturation and storage processes [22]. The protein has been identified as an early autoantibody target in type 1 diabetes [23, 24]. However, most recent findings showed that ZnT-8 is not exclusively expressed by pancreatic beta-cells, but also by pancreatic alpha-cells [25]. This fact disqualifies ZnT-8 as a potential candidate for noninvasive beta-cell imaging. If there was another purely beta-cell-specific marker, i.e. one that is exclusively expressed by pancreatic beta-cells, then ZnT-8 could be used additionally to monitor the fate of alpha-cells in disease and regimen conditions by subtracting the number of cells marked by the beta-cell-specific structure.
IC2 as the most promising probe applicable for noninvasive beta-cell imaging
IC2 is a rat IgM kappa monoclonal antibody generated from a B cell hybridoma. It binds to a sulfatide epitope galactose-3-sulphate present in the insulin granula. Though it was discovered almost 25 years ago [26], the antibody experienced a revival only recently after it had been proved as useful for beta-cell tracing in vivo [27, 28] due to its high specificity to these cells [29]. After intravenous administration, the IC2 antibody labeled with a radioisotope chelator specifically binds to beta-cell surface structures and can be detected by nuclear imaging. As the most specific beta-cell antibody, and the only currently available structure that binds exclusively to living insulin-producing beta-cells in the pancreas, it was recommended for future research by a board of beta-cell and imaging experts [28]. Despite the promising features of anti-IC2 monoclonal antibody, experimental and clinical studies verifying that it could be applied in human pancreas diagnostics are lacking so far.
Original findings using cellular ELISA showed an extraordinary high beta-cell specificity in studies on both primary rat cell (hepatocytes, fibroblast, lymphocytes, erythrocytes, macrophages and islet cells) and various other cell lines (liver BRL, lymphoid CL58, and the pancreatic beta-cell lines RIN-5F, RIN-5AH, INS-1E, MIN6 and HIT-T15) [26]. Furthermore, no staining has been detected on frozen sections of any tested tissue, and in particular not in the pancreas. Only live beta-cell in suspension expressed highly significant IC2 binding in fluorescence microscopy [26, 29, 30] and by fluorescent antibody cell sorter (FACS, Figure 3). Mouse, hamster and human islets expressed approximately 60% IC2-labeled cells, and these were only beta-cells.
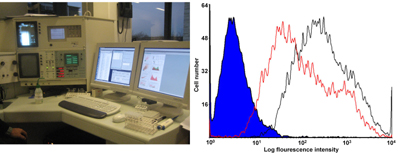 |
 |
Figure 3. Left: The image shows the Becton Dickenson FACS Vantage used in the routine analytical testing for IC2 autoantigen expression in vitro. This device is also applicable for specific preparative sorting of beta-cells from dispersed splitase/accutase dissociated islet cells. Right: Flow cytometric analysis with glucose sensitive RIN-5AH beta-cells stained indirectly at low glucose concentration (5 mM, red curve) and at high glucose concentration (12 mM, black curve) with IC2 followed by anti-rat IgM-Alexa 488. The blue top represents the isotype control rat IgM. Note that the 2 decades stronger binding of IC2 in comparison to control, with 1 decade increase in IC2 expression after glucose stimulation, is an indication of the functional state-dependent expression of the cell surface IC2 autoantigen. |
|
The target molecule is definitely not insulin, but is very likely to be a cell surface expressed insulin secretion-related molecule. The epitope for IC2 on functional and active beta-cells is probably also of conformational nature, highly sensitive to detergents and other denaturants. This makes it particularly difficult to identify and characterize the targeted autoantigen epitope, which might be of complex nature involving both a sulfatide (see above) and an anchoring plasma membrane protein (cellular binding is protease sensitive). It is possible that a member of the t-SNARE protein complex, which is expressed on the cell surface and involved in the regulation of insulin secretion, may be the epitope.
IC2 fulfills both the specificity and affinity criteria required for in vivo imaging (100 fold above background, see Figure 3 and [16]). Unfortunately, its large size and xenogenic rat IgM nature requires further fragmentation and engineering before clinical application for noninvasive beta-cell imaging may be successful. A study with fragmented antibodies applied for tumor imaging by microPET showed high affinity of the imaging probe to the target tissue [31]. In this study, antibodies were engineered in such a way that they maintained a high retention in tumor tissue combined with rapid blood clearance ability. Subsequently, the fragments were conjugated with a chelating agent (DOTA), i.v. administered and effectively imaged in the target tissue. Considering IC2, we know that rat IgM can easily be fragmented into smaller F(ab’)2 fragments, and recombinant fragments can even be generated with improved affinity through special mutational selection strategies. Chimeric or partly humanized rIC2 Fab or Fv fragments, if holding the same high affinity for human beta-cells, would be directly applicable for noninvasive clinical imaging aimed at estimating the functional beta-cells mass in vivo.
However, biodistribution studies in animal models of diabetes and in humans are still missing. These studies are urgently needed. The already proven in vitro specificity and in vivo homing exclusively to the beta-cells give us optimism for future clinical application, if sensitivity and specificity are high enough in humans and whole body imaging. DNA cloning, development of chimeric IC2, in vivo biodistribution studies and the obligatory toxicology testing are on the waiting list of urgent and necessary research for the development of a new generation beta-cell-specific contrast agent for noninvasive in vivo imaging. As yet, cytotoxic effects have not been detected in vitro, or in vivo, but more studies are needed concerning the impact of IC2 on insulin secretion on cellular level in vitro and in vivo.
Photoacoustic imaging as a novel modality for noninvasive beta-cell imaging
Photoacoustic tomography (PAT), also referred to variously as optoacoustic and thermoacoustic tomography, is a relatively new imaging technique that has gained attention in recent years due to its low-cost and competitive performance compared with conventional imaging modalities. The method relies on optical contrast but provides ultrasound-like resolutions based on light absorption by noble metal nanoparticles (Figure 4). Utilizing angiogenesis-driven optical absorption contrast, Manohar et al. applied near-infrared (NIR) photoacoustics for imaging breast cancer in human subjects [32] (Figure 5). However, the technique is still in its infancy and imaging of other human organs is only now being studied. Imaging the pancreas in humans may be difficult owing to its location behind the liver, which is a strongly absorbing organ.
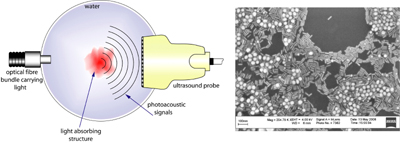 |
 |
Figure 4. Left: The concept of photoacoustic imaging, where absorption of pulsed near-infrared (NIR) light by an absorbing structure produces ultrasound which can be detected by conventional ultrasound detectors. Right: Scanning electron microscope (SEM) image of gold nanoparticles. The rod-shaped particles possess narrow and intense absorption bands in the NIR and when conjugated with IC2 antibodies can function as imaging probes for photoacoustic beta-cell imaging. |
|
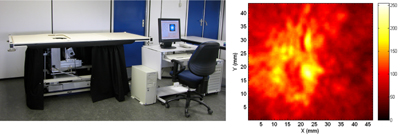 |
 |
Figure 5. Left: The Twente photoacoustic mammoscope, developed at the University of Twente, for the detection of tumors in the breasts of human patients. Right: Photoacoustic image of region-of-interest (ROI) of a patient's breast carrying a malignancy. The image is a slice at a depth of 12 mm from the breast surface. The ring-shaped structure marks the tumor invasion front with increased vascularization [25]. A miniature device producing similar images is under development for pancreas imaging in small animals. |
|
An area where PAT can make a considerable impact in diabetes research is in small-animal studies in basic research laboratories in academia and in the pharmaceutical industry for imaging mouse models of diabetes. These imaging studies can advance our fundamental understanding of diabetes onset and progression and also evaluate therapies. Small animal imagers based on photoacoustics have been unveiled by several groups [33-35], but as yet, studies on pancreas imaging have not been reported.
Research into sophisticated miniature imagers and an extensive knowledgebase on the use of gold nanoparticles [36-38], such as nanoshells and nanorods, bodes well for beta-cell imaging in the future. We envisage imaging probes for photoacoustics based on IC2 conjugated gold nanorods that can be used to study beta-cell loss and dysfunction in mouse models. Furthermore, this approach could permit monitoring of novel therapeutic approaches based on preservation or regeneration of beta-cells. This could lead to the definition of new roles for gold nanoparticle-based molecular photoacoustic imaging in fundamental diabetes research.
Conclusions
Noninvasive imaging of beta-cells and their function in vivo would be an important tool for evaluating the beta-cell mass in disease and therapy. The existing methods (MRI, PET, SPECT) still have drawbacks giving poor discrimination between the exocrine and endocrine pancreas, or a poor measurement of beta-cell mass. Most important, however, is the fact that none of these methods is able to work noninvasively in vivo without previous in vitro labeling of islet transplants. The only method of noninvasive beta-cell imaging is restricted to transplantation regimens using in vitro labeling with iron nanoparticles. Therefore, accurate assessment of the native beta-cell mass is still limited to autopsy studies. Monitoring of native beta-cells in vivo is not possible until today. The reason for this is the absence of appropriate targeting molecules with sufficient specificity to beta-cell-specific markers.
A new generation of targeting molecules is needed to developing imaging probes for monitoring the fate of transplanted or native beta-cells under various conditions in vivo. A specific biological structure, the IC2 monoclonal antibody, has shown enormous promise in initial studies. The great advantage of this structure is that it binds exclusively to beta-cells that are alive and insulin-producing. While the knowledge is available for a quantum leap in noninvasive imaging, much experimental work needs to be done to test this new technology and bring it to the clinic.
From the point of view of imaging science and technology, PAT is a new option that with the help of appropriate imaging probes has potential for imaging beta-cells in small animals [38]. Preliminary studies in animal models with tumors using noble metal nanorods conjugated with monoclonal antibodies appear promising [37]. With further technical improvement, PAT could develop into the method of choice for noninvasive imaging in small-animals for diabetes research.
Acknowledgments:
This work was supported by grant no. 00023001 from the Ministry of Health, Czech Republic, by the Danish Diabetes Society and the BioCampus Research Priority Area at University of Copenhagen, Denmark.
References
- Bonner-Weir S, Weir GC. New sources of pancreatic beta-cells. Nat Biotechnol 2005. 23:857-861. [DOD] [CrossRef]
- Meier JJ, Lin JC, Butler AE, Galasso R, Martinez DS, Butler PC. Direct evidence of attempted beta cell regeneration in an 89-year-old patient with recent-onset type 1 diabetes. Diabetologia 2006. 49:1838-1844. [DOD] [CrossRef]
- Ablamunits V, Sherry NA, Kushner JA, Herold KC. Autoimmunity and beta cell regeneration in mouse and human type 1 diabetes. The peace is not enough. Ann N Y Acad Sci 2007. 1103:19-32. [DOD] [CrossRef]
- Keymeulen B, Vandemeulebroucke E, Ziegler AG, Mathieu C, Kaufman L, Hale G, Gorus F, Goldman M, Walter M, Candon S, et al. Insulin needs after CD3-antibody therapy in new-onset type 1 diabetes. N Engl J Med 2005. 352(25):2598-2608. [DOD] [CrossRef]
- Saudek F, Havrdova T, Boucek P, Karasova L, Novota P, Skibova J. Polyclonal anti-T-cell therapy for type 1 diabetes mellitus of recent onset. Rev Diabet Stud 2004. 1(2):80-88. [DOD] [CrossRef]
- Pasquali L, Giannoukakis N, Trucco M. Induction of immune tolerance to facilitate beta cell regeneration in type 1 diabetes. Adv Drug Deliv Rev 2008. 60(2):106-113. [DOD] [CrossRef]
- Xu G, Stoffers DA, Habener JF, Bonner-Weir S. Exendin-4 stimulates both beta-cell replication and neogenesis, resulting in increased beta-cell mass and improved glucose tolerance in diabetic rats. Diabetes 1999. 48:2270-2276. [DOD] [CrossRef]
- Suarez-Pinzon WL, Lakey JR, Brand SJ, Rabinovitch A. Combination Therapy with Epidermal growth factor and gastrin induces neogenesis of human islet beta-cells from pancreatic duct cells and an increase in functional beta-cell mass. J Clin Endocrinol Metab 2005. 90(6):3401-3409. [DOD] [CrossRef]
- Pospisilik JA, Martin J, Doty T, Ehses J, Pamir N, Lynn FC, Piteau S, Demuth HU, McIntosh CH, Pederson RA. Dipeptidyl peptidase IV inhibitor treatment stimulates beta cell survival and islet neogenesis in streptozotocin-induced diabetic rats. Diabetes 2003. 52:741-750. [DOD] [CrossRef]
- Jirak D, Kriz J, Herynek V, Andersson B, Girman P, Burian M, Saudek F, Hajek M. MRI of transplanted pancreatic islets. Magn Reson Med 2004. 52:1228-1233. [DOD] [CrossRef]
- Berkova Z, Jirak D, Zacharovova K, Kriz J, Lodererova A, Girman P, Koblas T, Dovolilova E, Nebesarova J, Hajek M, Saudek F. Labeling of pancreatic islets with iron oxide nanoparticles for in vivo detection with magnetic resonance. Transplantation 2008. 85(1):155-159. [DOD]
- Kriz J, Jirak D, Girman P, Berkova Z, Zacharovova K, Honsova E, Lodererova A, Hajek M, Saudek F. Magnetic resonance imaging of pancreatic islets in tolerance and rejection. Transplantation 2005. 80:1596-1603. [DOD] [CrossRef]
- Toso C, Zaidi H, Morel P, Armanet M, Andres A, Pernin N, Baertschiger R, Slosman D, Buhler LH, Bosco D, Berney T. Positron-emission tomography imaging of early events after transplantation of islets of Langerhans. Transplantation 2005. 79(3):353-355. [DOD] [CrossRef]
- Eich T, Eriksson O, Lundgren T. Visualization of early engraftment in clinical islet transplantation by positronemission tomography. N Engl J Med 2007. 356(26):2754-2755. [DOD] [CrossRef]
- Toso C, Vallee JP, Ris F, Demuylder-Mischler S, Bosco D, Lepetit-Coiffe M, Saudek F, Shapiro AM, More P, Berney T. Clinical magnetic resonance imaging of pancreatic islet grafts after iron nanoparticle labelling. Am J Transplant 2008. 8(3):701-706. [DOD] [CrossRef]
- Sweet IR, Cook DL, Lernmark A, Greenbaum CJ, Wallen AR, Marcum ES, Stekhova SA, Krohn KA. Systematic screening of potential beta-cell imaging agents. Biochem Biophys Res Commun 2004. 314(4):976-983. [DOD] [CrossRef]
- Hampe CS, Wallen AR, Schlosser M, Ziegler M, Sweet IR. Quantitative evaluation of a monoclonal antibody and its fragment as potential markers for pancreatic beta cell mass. Exp Clin Endocrinol Diabetes 2005. 113(7):381-387. [DOD] [CrossRef]
- Maffei A, Liu Z, Witkowski P, Moschella F, Del Pozzo G, Liu E, Herold K, Winchester RJ, Hardy MA, Harris PE. Identification of tissue-restricted transcripts in human islets. Endocrinology 2004. 145(10):4513-4521. [DOD] [CrossRef]
- Noskova V, Bottalico B, Olsson H, Ehinger A, Pilka R, Casslen B, Hansson SR. Histamine uptake by human endometrial cells expressing the organic cation transporter EMT and the vesicular monoamine transporter-2. Mol Hum Reprod 2006. 12(8):483-489. [DOD] [CrossRef]
- Zanner R, Gratzl M, Prinz C. Circle of life of secretory vesicles in gastric enterochromaffin-like cells. Ann N Y Acad Sci 2002. 971:389-396. [DOD]
- Chimienti F, Devergnas S, Pattou F, Schuit F, Garcia-Cuenca R, Vandewalle B, Kerr-Conte J, Van Lommel L, Grunwald D, Favier A, Seve M. In vivo expression and functional characterization of the zinc transporter ZnT8 in glucose-induced insulin secretion. J Cell Sci 2006. 119(Pt 20):4199-4206. [DOD] [CrossRef]
- Chimienti F, Devergnas S, Favier A, Seve M. Identification and cloning of a beta-cell-specific zinc transporter, ZnT-8, localized into insulin secretory granules. Diabetes 2004. 53(9):2330-2337. [DOD] [CrossRef]
- Wenzlau JM, Juhl K, Yu L, Moua O, Sarkar SA, Gottlieb P, Rewers M, Eisenbarth GS, Jensen J, Davidson HW, Hutton JC. The cation efflux transporter ZnT8 (SLC30A8) is a major autoantigen in human type 1 diabetes. Proc Natl Acad Sci U S A 2007. 104(43):17040-17045. [DOD] [CrossRef]
- Gohlke H, Ferrari U, Koczwara K, Bonifacio E, Illig T, Ziegler AG. SLC30A8 (ZnT8) Polymorphism is Associated with Young Age at Type 1 Diabetes Onset. Rev Diabet Stud 2008. 5(1):23-26. [DOD]
- Gyulkhandanyan AV, Lu H, Lee SC, Bhattacharjee A, Wijesekara N, Fox JE, MacDonald PE, Chimienti F, Dai FF, Wheeler MB. Investigation of transport mechanisms and regulation of intracellular Zn2+ in pancreatic alpha-cells. J Biol Chem 2008. 283(15):10184-10197. [DOD] [CrossRef]
- Brogren CH, Hirsch F, Wood P, Druet P, Poussier P. Production and characterization of a monoclonal islet cell surface autoantibody from the BB rat. Diabetologia 1986. 29(5):330-333. [DOD] [CrossRef]
- Moore A, Bonner-Weir S, Weissleder R. Noninvasive in vivo measurement of beta-cell mass in mouse model of diabetes. Diabetes 2001. 50(10):2231-2236. [DOD] [CrossRef]
- Paty BW, Bonner-Weir S, Laughlin MR, McEwan AJ, Shapiro AM. Toward development of imaging modalities for islets after transplantation: insights from the National Institutes of Health Workshop on Beta Cell Imaging. Transplantation 2004. 77(8):1133-1137. [DOD] [CrossRef]
- Buschard K, Brogren CH, Ropke C, Rygaard J. Antigen expression of the pancreatic beta-cell is dependent on their functional state, as shown by a specific, BB rat monoclonal autoantibody IC2. APMIS 1988. 96(4):342-346. [DOD]
- Aaen K, Rygaard J, Josefsen K, Petersen H, Brogren CH, Horn T, Buschard K. Dependence of antigen expression on functional state of beta-cells. Diabetes 1990. 39 (4):697-701. [DOD] [CrossRef]
- Wu AM, Yazaki PJ, Tsai S, Nguyen K, Anderson AL, McCarthy DW, Welch MJ, Shively JE, Williams LE, Raubitschek AA, et al. High-resolution microPET imaging of carcinoembryonic antigen-positive xenografts by using a copper-64-labeled engineered antibody fragment. Proc Natl Acad Sci U S A 2000. 97(15):8495-8500. [DOD] [CrossRef]
- Kruger RA, Kiser WL, Reinecke DR, Kruger GA, Miller KD. Thermoacoustic optical molecular imaging of small animals. Mol Imaging 2003. 2(2):113-123. [DOD] [CrossRef]
- Wang X, Pang Y, Ku G, Xie X, Stoica G, Wang LV. Noninvasive laser-induced photoacoustic tomography for structural and functional imaging of the brain in vivo. Nat Biotechnol 2003. 21(7):803-806. [DOD] [CrossRef]
- Siphanto RI, Thumma KK, Kolkman RG, Van Leeuwen TG, De Mul FF, Van Neck JW, Van Adrichem LN, Steenbergen W. Serial noninvasive photoacoustic imaging of neovascularization in tumor angiogenesis. Opt Express 2005. 13:89. [DOD] [CrossRef]
- Manohar S, Vaartjes SE, Van Hespen JC, Klaase JM, Van den Engh FM, Steenbergen W, Van Leeuwen TG. Initial results of in vivo non-invasive detection of cancer in the human breast using near-infrared photoacoustic imaging. Opt Express 2007. 15:12277-12285. [DOD] [CrossRef]
- Wang Y, Xie X, Wang X, Ku G, Gill KL, O'Neal DP, Stoica G, Wang LH. Photoacoustic tomography of a nanoshell contrast agent in the in vivo rat brain. Nano Lett 2004. 4:1689. [DOD] [CrossRef]
- Eghtedari M, Oraevsky A, Copland JA, Kotov NA, Conjusteau A, Motamedi M. High sensitivity of in vivo detection of gold nanorods using a laser optoacoustic imaging system. Nano Lett 2007. 7(7):1914-1918. [DOD] [CrossRef]
- Rayavarapu RG, Petersen W, Ungureanu C, Post JN, van Leeuwen TG, Manohar S. Synthesis and bioconjugation of gold nanoparticles as potential molecular probes for light-based imaging techniques. Int J Biomed Imaging 2007. 2007:29817. [DOD]
This article has been cited by other articles:
|
Human islet cell MORF/cMORF pretargeting in a xenogeneic murine transplant model
Liu G, Dou S, Cheng D, Leif J, Rusckowski M, Streeter PR, Shultz LD, Hnatowich DJ, Greiner DL
Mol Pharm 2011. 8(3):767-773
|
|
|
Type 1 diabetes: etiology, immunology, and therapeutic strategies
Van Belle TL, Coppieters KT, Von Herrath MG
Physiol Rev 2011. 91(1):79-118
|
|
|
Assessment of human islet labeling with clinical grade iron nanoparticles prior to transplantation for graft monitoring by MRI
Ris F, Lepetit-Coiffe M, Meda P, Crowe LA, Toso C, Armanet M, Niclauss N, Parnaud G, Giovannoni L, Bosco D, Morel P, Vallee JP, Berney T
Cell Transplant 2010. 19(12):1573-1585
|
|
|
Pancreatic beta cell mass PET imaging and quantification with [(11)C]DTBZ and [ (18)F]FP-(+)-DTBZ in rodent models of diabetes
Singhal T, Ding YS, Weinzimmer D, Normandin MD, Labaree D, Ropchan J, Nabulsi N, Lin SF, Skaddan MB, Soeller WC, Huang Y, Carson RE, Treadway JL, Cline GW
Mol Imaging Biol 2010. Sep 8, epub ahead of print
|
|
|
Rat pancreas uptake of (11)C dihydrotetrabenazine stereoisomers
Kilbourn MR
Nucl Med Biol 2010. 37(8):869-871
|
|
|
Preparation of (111)In-DTPA morpholino oligomer for low abdominal accumulation
Liu G, Dou S, Rusckowski M, Greiner D, Hnatowich D
Appl Radiat Isot 2010. 68(9):1709-1714
|
|
|
The role of transmembrane protein 27 (TMEM27) in islet physiology and its potential use as a beta cell mass biomarker
Altirriba J, Gasa R, Casas S, Ramirez-Bajo MJ, Ros S, Gutierrez-Dalmau A, Ruiz de Villa MC, Barbera A, Gomis R
Diabetologia 2010. 53(7):1406-1414
|
|
|
Imaging Pancreatic Beta-Cells: Update from the 4th Workshop of the National Institutes of Health, Washington DC, April 2009
Ris F, Crowe L, Berney T
Rev Diabet Stud 2009. 6(4):279-282
|
|
|
Mouse models for type 1 diabetes
Van Belle TL, Taylor P, von Herrath MG
Drug Discov Today Dis Models 2009. 6(2):41-45
|
|
|
Noninvasive imaging of pancreatic beta cells
Malaisse WJ, Louchami K, Sener A
Nat Rev Endocrinol 2009. 5(7):394-400
|
|
|
Intraindividual comparison of 68Ga-DOTA-TATE and 18F-DOPA PET in patients with well-differentiated metastatic neuroendocrine tumours
Haug A, Auernhammer CJ, Wängler B, Tiling R, Schmidt G, Göke B, Bartenstein P, Pöpperl G
Eur J Nucl Med Mol Imaging 2009. 36(5):765-770
|
|
|
Zinc signalling and subcellular distribution: emerging targets in type 2 diabetes
Mocchegiani E, Giacconi R, Malavolta M
Trends Mol Med 2008. 14(10):419-428
|
|
|