Original Data
Rev Diabet Stud,
2010,
7(1):62-73 |
DOI 10.1900/RDS.2010.7.62 |
The Humanized NOD/SCID Mouse as a Preclinical Model to Study the Fate of Encapsulated Human Islets
Vijayaganapathy Vaithilingam1,2, Jose Oberholzer3, Gilles J. Guillemin4, Bernard E. Tuch1,2,5
1Diabetes Transplant Unit, Prince of Wales Hospital and University of New South Wales, and Australian Foundation for Diabetes Research, Sydney, Australia
2Australian Foundation for Diabetes Research, Sydney, Australia
3Department of Surgery, University of Illinois at Chicago, USA
4Department of Pharmacology, University of New South Wales, Australia
5Now at Division of Materials, Science and Engineering, Commonwealth Scientific and Industrial Research Organization, Sydney, Australia
Address correspondence to: Bernard E. Tuch, e-mail: bernie.tuch@csiro.au
Manuscript submitted April 16, 2010; resubmitted May 3, 2010; accepted May 5, 2010.
Keywords: diabetes, NOD/SCID mouse, alloreactivity, cytokines, chemokines, immunoregulation, fibrosis, humanized mouse, microencapsulated islets, transplantation
Abstract
Despite encouraging results in animal models, the transplantation of microencapsulated islets into humans has not yet reached the therapeutic level. Recent clinical trials using microencapsulated human islets in barium alginate showed the presence of dense fibrotic overgrowth around the microcapsules with no viable islets. The major reason for this is limited understanding of what occurs when encapsulated human islets are allografted. This warrants the need for a suitable small animal model. In this study, we investigated the usefulness of NOD/SCID mice reconstituted with human PBMCs (called humanized NOD/SCID mice) as a preclinical model. In this model, human T cell engraftment could be achieved, and CD45+ cells were observed in the spleen and peripheral blood. Though the engrafted T cells caused a small fibrotic overgrowth around the microencapsulated human islets, this failed to stop the encapsulated islets from functioning in the diabetic recipient mice. The ability of encapsulated islets to survive in this mouse model might partly be attributed to the presence of Th2 cytokines IL-4 and IL-10, which are known to induce graft tolerance. In conclusion, this study showed that the hu-NOD/SCID mouse is not a suitable preclinical model to study the allograft rejection mechanisms of encapsulated human islets. As another result, the maintained viability of transplanted islets on the NOD/SCID background emphasized a critical role of protective mechanisms in autoimmune diabetes transplanted subjects due to specific immunoregulatory effects provided by IL-4 and IL-10.
Abbreviations: APC - allophycocyanin; BGL - blood glucose level; BSA - bovine serum albumin; CD3 - cluster of differentiation 3 (glycoprotein expressed on T cells for signal transduction); CD4 - cluster of differentiation 4 (glycoprotein expressed on Th, Treg, and dendritic cells; co-receptor assisting the T cell receptor); CD8 - cluster of differentiation 8 (glycoprotein expressed on T cells; co-receptor for the T cell receptor); CD19 - cluster of differentiation 19 (protein expressed on expressed on dendritic and B cells); CD45 - cluster of differentiation 45 (tyrosinphosphatase; protein expressed on almost all blood cells incl. B cells); 6-CFDA - 6-carboxyfluorescein diacetate; CMRL - Connaught medical research labs; CO2 - carbon-dioxide; CRML-1066 - cell culture medium (to maintain growth of permanent cell lines); FACS - fluorescence activated cell sorting; FITC - fluorescein isothiocyanate; GAD - glutamic acid decarboxylase; HBSS - Hanks' balanced salt solution; hu - human; ICA512 - islet cell antigen 512 (autoantigen of type 1 diabetes); IEQ - islet equivalent number; IFN-γ - interferon gamma; IL - interleukin; IP-10 - inducible protein of 10 kD (chemokine; also called C-X-C motif ligand 10 (CXCL10)); MCP-1 - monocyte chemoattractant protein-1; MIP - macrophage inflammatory protein; NaCl - sodium chloride; NOD - nonobese diabetic; OGTT - oral glucose tolerance test; PBMC - peripheral blood mononuclear cell; PBS - phosphate buffered saline; PE - phycoerythrin (fluorescence dye for labeling antibodies); PI - propidium iodide; RANTES - regulated on activation, normal T cell expressed and secreted (chemokine; chemoattractant for monocytes and T cells); RIA - radioimmunoassay; SCID - severe combined immunodeficiency (model without functional T and B cells); TCR - T cell receptor; Th cell - T helper cell; TNF-α - tumor necrose factor α
Introduction
Microencapsulating human islets is a means to overcome the immune-mediated rejection of the graft without toxic immunosuppression. However, the method is not yet fully developed and still under investigation due to early loss of islet viability. Recently, phase 1 clinical trials using calcium alginate-poly-L-ornithine [1] and barium alginate microcapsules [2] showed that allografting of microencapsulated human islets is safe although only a minor clinical benefit was observed. Laparoscopic retrieval of the microcapsules showed the presence of dense fibrotic overgrowth around microcapsules with no viable islets [2]. The reasons for this impairment are not clear. Possible factors being considered are inflammatory response initiated in response to antigens released from the encapsulated islets, and hypoxic conditions of the graft site [3, 4]. An understanding of these mechanisms should result in improvements in future clinical trials. In an attempt to obtain such knowledge, we have transplanted encapsulated human islets into the so called "humanized mouse".
The humanized mouse is an immunodeficient mouse that has received human hematopoietic cells. Whilst a number of models have been developed [5-8], we have chosen to use the NOD/SCID mouse injected intraperitoneally with peripheral blood mononuclear cells (PBMCs). This animal model is called the humanized NOD/SCID (hu-NOD/SCID) mouse. We have used this model previously and shown its suitability to cause rejection of maturing beta-cells [9]. In this model, PBMC circulate through the animal and can be found in spleen, bone marrow, blood, and other organs. An advantage of this model is that cellular rejection with T cells occurs. In some other models engraftment could be achieved, but most of the human cells are B lymphocytes [10].
To date, humanized mice have not been used to study the fate of engrafted microencapsulated human islets. This study aimed to evaluate the suitability of hu-NOD/SCID mice as a preclinical model for the study of the fate of microencapsulated transplanted islets.
Materials and methods
Human islet isolation and shipment
Human islets were isolated from pancreases of deceased multiorgan donors (n = 3) at the Cell Isolation Laboratory at the University of Illinois at Chicago, USA. Briefly, human islets were isolated by digestion with Serva Collagenase NB 1 (Nordmark, Germany) using the method described previously [11]. The isolated human islets were then cultured in supplemented CMRL-1066 media (Mediatech, Herndon, VA) containing 1.5% human albumin for 1-2 days before being shipped to Sydney using a commercial courier service. The islets were then cultured for a day before being encapsulated. All procedures associated with the extraction and preparation of human islets were approved by the institution’s Human Research Ethics Committee.
Microencapsulation
The cultured human islets were pooled together and washed in 0.9% NaCl. The human islets were then suspended in highly purified 2.2% alginate (60:40, glucuronic acid:mannuronic acid, UPMVG PRONOVA, FMC Biopolymer) solution in 1:8 ratio. The microcapsule formation was carried out in an air-driven droplet generator (Steinau, Berlin, Germany), as described previously [12]. The microcapsules formed were incubated in a 20 mM barium chloride precipitation bath for 2 min. After gelation, the microcapsules were washed thrice in 0.9% NaCl to remove excess barium. The average size of the microcapsules was 560 µm (range 500-700 µm, median -550 µm). The microencapsulated human islets were then cultured for a day in CMRL-1066 media prior to in vivo studies.
Animals
Both non-diabetic and diabetic female NOD/SCID (6-8 weeks) mice were used in this study. The animals were made diabetic by three consecutive intraperitoneal injections of streptozotocin (70 mg/kg, Alexis Biochemicals). Animals with three consecutive blood glucose levels >12 mmol/l were considered diabetic and used for the study. The animals were divided into three groups: i) controls (non-diabetic non-humanized mice) killed at day 14, ii) non-diabetic humanized mice killed at days 1, 7, and 14 post-humanization, and iii) diabetic humanized mice killed at day 23 post-humanization.
Transplantation of microencapsulated human islets
The mice were anesthetized by intraperitoneal injection of pentobarbitone (10 mg/ml) at a dose of 65 mg/kg. The encapsulated human islets were then injected into the peritoneal cavity using a 20-gauge catheter. The incision site was sutured and stapled. All the three groups of mice were transplanted with 3,000 IEQs of encapsulated islets with a graft volume of 500 µl. All the procedures involving the mice were approved by the Institution's Animal Care and Ethics Committee
Humanization of mice
One week after diabetic and non-diabetic NOD/SCID mice had been transplanted with 3,000 IEQs of encapsulated human islets, they were reconstituted with human immune cells. Human PBMCs were obtained from whole blood preparations by Lymphoprep Ficoll density gradient centrifugation (Axis-Shield PoC AS, Oslo, Norway). The isolated PBMCs were then washed in phosphate buffered saline (PBS), and resuspended in PBS at a concentration of 5 × 107 cells/ml. Subsequently, PBMCs were injected intraperitoneally into the transplanted NOD/SCID mice. The PBMCs were isolated from non-diabetic volunteers who were tested negative for autoantibodies against the islet cell antigens, ICA512 and GAD. All the procedures associated with the extraction and preparation of human blood were approved by the institution's Human Research Ethics Committee.
Monitoring of mice
Body weight and blood glucose level (BGL) were measured daily for all mice in each of the three groups of mice. BGL was measured using a portable glucometer (Accu-Chek Advantage, Roche, Germany). Diabetic NOD/SCID mice were considered normoglycemic after three consecutive BGL readings of ≤ 6 mmol/l.
Oral glucose tolerance test
To evaluate graft function, an oral glucose tolerance test (OGTT) was performed in diabetic humanized NOD/SCID mice at 21 days post-humanization. For this, the mice were fasted overnight followed by an oral glucose gavage (3 mg/g of 300 mg/ml glucose solution) and BGL was measured at 0, 20, 40, 60, and 120 minutes after glucose administration. OGTT was also carried out on diabetic and non-diabetic non-humanized control NOD/SCID mice.
Graft retrieval and collection of peritoneal fluid
The non-diabetic humanized and non-humanized mice were maintained for different time points (1, 7, and 14 days) post-humanization. The experiments terminated when the mice were killed by carbon-dioxide (CO2). Capsules were recovered from animals by peritoneal lavage with PBS containing 1% bovine serum albumin (BSA). The microcapsules were removed from the peritoneal lavage fluid by gravity separation. The resulting peritoneal fluid was then spun at 3,000 rpm to remove immune cells and debris, and then frozen at -80ºC for future analysis of chemokines/cytokines. The peritoneal cavity was then flushed with 0.9% NaCl and subsequently examined for any remaining capsules. The volume of graft recovered from each animal was recorded. Capsules were observed under the microscope for evidence of breakage (%) and fibrotic overgrowth. For the latter we used the following scoring system: 0 = no overgrowth, 1 = <25% of capsule overgrown, 2 = 25-50% of capsule overgrown, 3 = 50-75% capsule overgrown and 4 = >75% overgrown), see Figure 1. A random aliquot of capsules were then taken for viability and static stimulation studies.
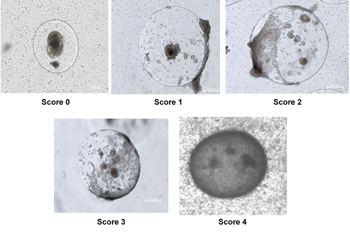 |
 |
Figure 1. Scoring system used to assess the degree of fibrotic overgrowth. The microcapsules retrieved from non-diabetic humanized mice were observed under microscope for the presence of fibrotic overgrowth and assessed. The degree of fibrotic overgrowth was measured based on the following scoring system: score 0 = no overgrowth, score 1 = <25 % overgrowth, score 2 = >25 % <50 % overgrowth, score 3 = >50 % <70 % overgrowth, score 4 = >75 % overgrowth. |
|
Viability
Viability of the retrieved encapsulated human islets from both non-diabetic humanized and non-humanized mice was assessed using the fluorescent dyes 6-carboxyfluorescein diacetate (6-CFDA; Sigma, St. Louis, MO) and propidium iodide (PI; Sigma). The percentage of green (live cells) to red (dead cells) fluoresence was assessed to evaluate the viability (n = 100, for each time point and each mice). Samples were analyzed under a Zeiss-Axioskop 2 microscope using Axiovision LE software.
Fluorescence-activated cell-sorting analysis
1, 7, and 14 days after PBMC injection, spleen, bone marrow, and peripheral blood were recovered from both non-diabetic humanized and non-humanized mice. Single-cell suspensions of the same mice were prepared by mechanical disruption. Erythrocytes in the preparations were lysed using 10% fluorescence-activated cell-sorting (FACS) lysing solution (BD Biosciences, Sydney, Australia). The following antibodies purchased from BD Biosciences were used for staining: anti-mouse CD45 fluorescein isothiocyanate (FITC)-conjugated (clone 30-F11), anti-human CD45 allophycocyanin (APC)-conjugated (clone HI30), anti-human CD19 phycoethrin (PE)-conjugated (clone HIB19), and the MultiTEST CD3 FITC/CD8 PE/CD45 PerCP/CD4 APC kit. Single-cell suspensions were incubated with the relevant antibodies for 30 min and analyzed using a FACScaliburII sorter and Cell Quest FACS analysis system (BD Biosciences). Approximately 50,000 events were analyzed per sample. The cell suspensions stained with the respective isotype control for each antibody were used as negative controls. The engraftment of human CD45+ cells in the analyzed tissues was calculated as the percentage of the total number of CD45+ cells (mouse plus human) detected.
Quantification of chemokines and cytokines in the peritoneal fluid
Quantification of different chemokines and cytokines was measured with Luminex technology using a human Lincoplex cytokine kit (Linco Research, Missouri, USA), according to the manufacturer’s protocol. Cytokines analyzed were both T-helper 1 (Th1) cell-related (IL-2, IL-5, IL-12, IL-1β, IL-1RA, IFN-γ, and TNF-α), and Th2-related (IL-4, IL-10). Analyzed chemokines were MCP-1, MIP-1α, MIP-1β, IP-10, eotaxin and RANTES. Briefly, antibody-coated cytokine-specific beads were added to 96 well Millipore plates (Millipore). Peritoneal fluid (25 µl) collected at days 1, 7, and 14 (from both non-diabetic humanized and non-humanized mice) were added and incubated for 1 hour at room temperature with mild agitation, allowing cytokines to bind to the cytokine-specific beads. Subsequently, plates were washed and incubated with biotinylated anti-cytokine detection antibody for 30min. After washing, streptavidin PE was added for 30min to bind to the detection antibody. Fluorescence labeling of cytokine-specific beads was analyzed by a double-laser Bio-plex reader (Bio-Rad, Australia). Cytokine concentration was determined based on a standard curve included in each plate, using cytokine standards provided by the manufacturer.
Insulin secretion
Aliquots of encapsulated human islets retrieved from both non-diabetic humanized and non-humanized mice at days 1, 7, and 14 were exposed to either 2.8 mM glucose (basal, n = 3) or 20 mM glucose (stimulus, n = 3) for one hour at 37ºC. After 1 hour, the supernatant was collected and human insulin measured by radioimmunoassay (RIA; Linco). The remaining pellet was washed in Hanks' balanced salt solution (HBSS) solution followed by addition of cold acid ethanol and vortexed vigorously to enhance cell lysis. The cell extract was kept at 4ºC overnight and the supernatant collected the following day for measuring insulin content by RIA.
Human C-peptide assay
Serum samples were collected from recipient mice and stored at -20°C until analysis. The levels of human C-peptide were quantified using Linco human C-peptide RIA kit (Linco research, Missouri, USA) according to the manufacturer’s instructions.
Statistical analysis
All values were expressed as mean ± SEM and the statistical package NCSS97 was used to perform the statistical analysis. Standard Student's t-test was used to compare the data between the two groups. One-way analysis of variance (Duncan's multiple comparison test) was used to compare data in more than two groups. Differences among groups were considered significant if p < 0.05.
Results
Engraftment of human T cells in non-diabetic hu-NOD/SCID mice
Non-diabetic NOD/SCID mice transplanted with 3,000 IEQs of encapsulated human islets were reconstituted with human PBMCs (5 × 107 cells/ml). Non-humanized mice receiving the same number of IEQs were used as controls. To confirm the engraftment of human PBMCs in NOD/SCID mice, the human CD45+ cells and its subsets were analyzed in the spleen, peripheral blood and bone marrow by flow cytometry at days 1, 7, and 14 post-humanization. As seen in Figure 2, mice at days 1, 7, and 14 had similar levels of CD45+ cell engraftment in spleen (37.1 ± 17.1, 42.2 ± 19.4, and 42.5 ± 19.8 %), peripheral blood (16.9 ± 9.7, 21.6 ± 14.7, and 19.7 ± 12 %), and bone marrow (0.4 ± 0.2, 0.6 ± 0.2, and 0.4 ± 0.2 %), respectively. No human T cells were detected in the tissues of non-humanized controls (Figure 2). The subsets of CD45+ cells were analyzed by FACS staining for a B cell (CD19), cytotoxic T cell (CD8), and helper T cell (CD4) marker. We found that the proportion of these cells in spleen, blood, and bone marrow was not significantly different between days 1, 7, and 14 post-humanization (Table 1). CD45+ cells infiltrating these organs consisted mostly of CD8+ and CD4+ T cells with low number of CD19+ B cells. On the other hand, no human CD45+ cell subsets were detected in the bone marrow at days 1, 7, and 14 (Table 1).
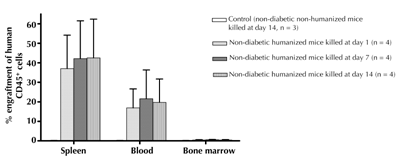 |
 |
Figure 2. Flow cytometry analysis for the engraftment of human CD45+ cells in non-diabetic humanized mice. Non-diabetic NOD/SCID mice were transplanted with 3,000 IEQs of encapsulated human islets intra-peritoneal. One week after transplantation mice were humanized by injecting with 5 × 107cells/ml of human PBMCs intra-peritoneal. The mice were killed at days 1, 7, and 14 post-humanization, and engraftment of human CD45+ cells in spleen, blood, and bone marrow were assessed. Humanized mice at days 1, 7, and 14 had similar levels of human CD45+ cell engraftment in spleen, peripheral blood, and bone marrow (p > 0.05). No human T cells were detected in the tissues of non-humanized controls. Controls were non-diabetic non-humanized mice transplanted with 3,000 IEQs of encapsulated human islets killed at day 14. Values are mean ± SEM. |
|
Table
1.
Flow cytometry analysis for human CD45+ cell subset engraftment in non-diabetic humanized mice |
|
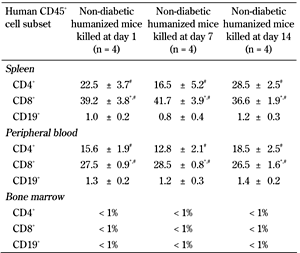 |
 |
Legend:
Data indicated in percentage. Subsets of human CD45+ cells in spleen, blood, and bone marrow of non-diabetic humanized mice were determined using specific antibodies against cytotoxic CD8+ T cells, helper CD4+ T cells, and CD19+ B cells. The CD45+ cells consisted mostly of CD8+ and CD4+ T cells with low number of CD19+ B cells at days 1, 7, and 14 post-humanization. The levels of CD8+ T cells were higher both in spleen and blood compared with CD4+ T cells (p < 0.05). No human CD45+ cell subsets were detected in the bone marrow at days 1, 7, and 14. Values = mean ± SEM. * p < 0.05, days 1, 7, and 14. Spleen and peripheral blood: CD8+ > CD4+. # p < 0.05, days 1, 7, and 14. Spleen and peripheral blood: CD8+ and CD4+ > CD19+. |
|
Evaluation of the retrieved microencapsulated islets from non-diabetic hu-NOD/SCID mice
Non-diabetic hu-NOD/SCID mice were killed at days 1, 7, and 14 post-humanization, and the grafts were retrieved. Non-humanized mice receiving the same number of IEQs were used as controls. The viability of grafted encapsulated islets decreased slightly, but significantly, in humanized mice on days 7 (77.2 ± 0.6 %) and 14 (75.5 ± 0.6 %), respectively (p < 0.05). This decrease was not yet evident at day 1 (81.04 ± 0.5 %) compared with non-humanized controls at day 14 (81.9 ± 0.7 %) (Figure 3). Fibrotic overgrowth was seen around microcapsules retrieved on days 7 and 14, but not at day 1 in humanized mice. The degree of fibrotic overgrowth was higher on day 14 compared with day 7; with score 2 (21.0 ± 0.9 vs. 16.3 ± 0.9 %), and score 3 (13.5 ± 1.7 vs. 8.1 ± 1 %), respectively (Figure 3 and Table 2). No fibrotic overgrowth was evident on the microcapsules retrieved from non-humanized controls at day 14. There was no difference in the percentage of retrieved and intact capsules indicating that the microcapsules were free floating, biocompatible, and intact (Table 2).
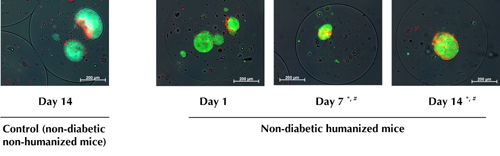 |
 |
Figure 3. Viability of encapsulated human islets retrieved from non-diabetic humanized mice killed at different time points as assessed by CFDA and PI staining. Viability of human islets dropped significantly at days 7 and 14 retrieved from non-diabetic humanized mice compared with non-diabetic humanized mice at day 1 and controls. Controls were encapsulated human islets retrieved from non-diabetic non-humanized mice killed at day 14 post-humanization. *, # p < 0.05, n = 4, each time point. * Non-humanized mice > humanized mice at days 7 and 14. # Humanized mice day 1 > humanized mice at days 7 and 14. *, # ANOVA and Duncan’s multiple comparison test. |
|
Table
2.
Evaluation of the retrieved microencapsulated human islets from non-diabetic humanized mice |
|
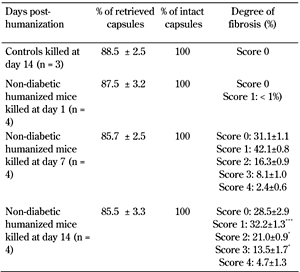 |
 |
Legend:
Values are percent ± SEM. Controls are non-diabetic non humanized mice. Non-diabetic mice transplanted with 3,000 IEQs of encapsulated human islets were humanized by injecting with 5 × 107 cells/ml of human PBMCs. The mice were killed at days 1, 7, and 14 post-humanization and the microcapsules retrieved. The microcapsules were assessed for fibrotic overgrowth using a scoring system. The degree of fibrotic overgrowth was higher on day 14 compared with day 7. *** p < 0.001, score 1 (day 7 vs. day 14). * p < 0.05, score 2 and 3 (day 7 vs. day 14), t-test. |
|
Concomitant with the presence of fibrotic overgrowth there was significantly less serum C-peptide levels in humanized mice on days 7 (1.7 ± 0.1 ng/ml) and 14 (1.4 ± 0.1 ng/ml) compared with day 1 and control (Table 3). Also, there was no significant difference in glucose-induced insulin secretion in the retrieved microencapsulated human islets from both humanized and non-humanized mice on day 14; indicating that the graft was still functional (Figure 4).
Table
3.
Blood glucose and human C-peptide levels of non-diabetic humanized mice |
|
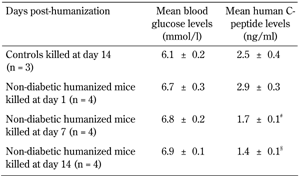 |
 |
Legend:
Values are percent ± SEM. Controls are non-diabetic non humanized mice. Non-diabetic mice transplanted with 3,000 IEQs of encapsulated human islets were humanized by injecting with 5 × 107 cells/ml of human PBMCs. Mice were killed at days 1, 7, and 14 post-humanization, and the human C-peptide levels measured. There were significantly less serum C-peptide levels in humanized mice on days 7 and 14 compared with day 1 and controls. # Non-diabetic humanized mice at day 7 < control (non-diabetic non-humanized mice day 14), and non-diabetic humanized mice at day 1. § Non-diabetic humanized mice at day 14 < control (non-diabetic non-humanized mice day 14), and non-diabetic humanized mice at day 1. #, § ANOVA and Duncan’s multiple comparison test. |
|
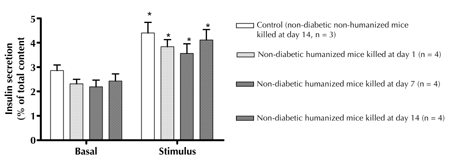 |
 |
Figure 4. Static stimulation studies of encapsulated human islets retrieved from non-diabetic humanized mice. Basal (2.8 mM) and glucose-stimulated (20 mM) insulin secretion of retrieved microencapsulated human islets at days 1, 7, and 14 from non-diabetic humanized mice behaved in a similar manner to those retrieved from controls (non-diabetic non-humanized mice) at day 14, indicating the graft was still functional. Values = mean ± SEM. * p < 0.05, basal vs. stimulus, t-test. |
|
Evaluation of chemokines and cytokines in the peritoneal fluid of non-diabetic hu-NOD/SCID mice
Activated T cells can produce many cytokines. Analyzing these cytokines in the humanized mice transplanted with human islets should assist in understanding the nature of the immune response. Th2 cyotkines IL-4 and IL-10 were present in the peritoneal fluid of grafted humanized mice at all time points, but not in the non-humanized controls (Table 4). In contrast, Th1-related cytokines were undetectable, except IFN-γ, and then only at day 14 at levels barely detectable.
Table
4.
Evaluation of chemokines and cytokines in the peritoneal fluid of non-diabetic humanized mice |
|
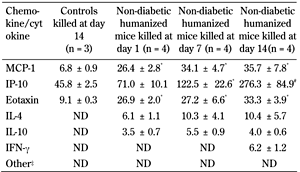 |
 |
Legend:
Values are mean (pg/ml) ± SEM. Controls are non-diabetic non-humanized mice. Th2 cytokines (IL-4 and IL-10) rather than Th1 were present in the peritoneal fluid of grafted humanized mice at all time points, but not in the non-humanized controls. The lower limit of the assay was 3.2 pg/ml. ‡ Other include MIP-1α, MIP-1β, RANTES, TNF-α, IL-1β, IL-2, IL-5, IL-12, IL-1RA. * p < 0.05, controls < non-diabetic humanized mice killed at days 1, 7, and 14. # p < 0.05, controls and non-diabetic humanized mice killed at day 1 < non-diabetic humanized mice killed at day 14. *, # ANOVA and Duncan's multiple comparison test. ND: not detectable. |
|
Of the six chemokines measured, three were detectable (MCP-1, eotaxin, and IP-10) in both the humanized and non-humanized grafted mice, but levels were much higher in the humanized animals. There was no significant difference in the level of chemokines in the humanized mice at days 1, 7, and 14 post-humanization except for IP-10 (Table 4). The presence of these chemokines (MCP-1, eotaxin, and IP-10) in the non-humanized mice suggest that human islets might be their source as they could not be detected in peritoneal fluid of non-humanized mice transplanted with empty capsules (data not shown).
Encapsulated human islets restore euglycemia in diabetic hu-NOD/SCID mice
To determine the fate of encapsulated human islets in diabetic hu-NOD/SCID mice, 3,000 IEQs of encapsulated human islets were transplanted intraperitoneally into streptozotocin-induced diabetic NOD/SCID mice. All mice became euglycemic by 2.5 ± 0.5 days, with the BGL declining from 17.5 ± 1.1 to 4.7 ± 0.5 mmol/l (Figure 5A). After 7 days of stable euglycemia, the mice were humanized by an intraperitoneal injection of 5 × 107 cells/ml human PBMCs. The animals were monitored for a further 23 days to determine graft fate. As shown in Figure 5A, grafts survived and continued to function, with mice remaining euglycemic. To assess graft function further, an OGTT was carried out at 21 days post-humanization. All mice transplanted with 3,000 IEQs handled glucose normally and exhibited blood glucose levels similar to non-diabetic non-humanized controls (Figure 5B).
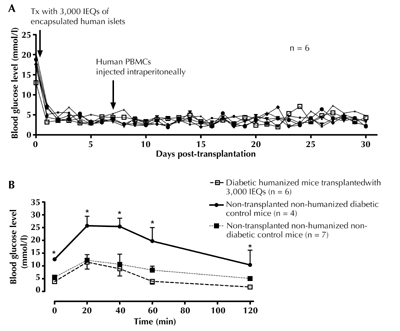 |
 |
Figure 5. Transplantation of encapsulated human islets into diabetic humanized mice. 3,000 IEQs of encapsulated human islets were transplanted into peritoneal cavity of female NOD/SCID mice rendered diabetic by streptozotocin (n = 6). Normoglycemia was achieved in all mice by 2.5 ± 0.5 days post-transplantation. 7 days after normalization of blood glucose levels mice were humanized by injecting 5 × 107 cells/ml of human PBMCs intraperitoneal. A: There was no evident graft rejection till 23 days post-humanization, and all mice remained euglycemic till the end of experiment. B: Oral glucose tolerance test at 21 days post-humanization showed that the grafts were still functional. Values are mean ± SEM. * p < 0.05, diabetic humanized mice and non-diabetic non-humanized control < non-humanized diabetic control (ANOVA and Duncan’s multiple comparison test). |
|
Discussion
Many humanized mice models have been used previously to study the fate of transplanted islets [5-8]. However, none of these models have been employed to study the mechanisms of fibrotic overgrowth of microencapsulated human islets after transplantation. In this study, we investigated the suitability of human PBMC reconstituted mouse model, namely the hu-NOD/SCID mouse, to affect transplanted encapsulated islets. Encapsulated islets retrieved from non-diabetic humanized mice on days 7 and 14 showed the presence of fibrotic overgrowth with a significant drop in viability and C-peptide levels compared with day 1 and non-humanized controls (Table 2, Figure 2, and Table 3). However, human C-peptide was still detectable in mouse serum on day 14 (Table 3), and retrieved encapsulated islets were glucose responsive (Figure 4). Moreover, there was no necrosis evident in the islets. These results show that the human PBMCs had little adverse effects, and that there was sufficient nutrient supply for the encapsulated islets to function in a reasonable manner. This outcome is very different from that observed when encapsulated islets were allografted by us into the peritoneal cavity of diabetic humans [2]. The lack of adverse results in the mice cannot be due to poor immune reconstitution, since rejection of islets and other allografted tissue rejection has been shown to occur in similar models with comparable levels of human PBMCs engraftment [5, 6, 8]. Previously, we have successfully used a similar mouse model to study the allograft rejection of human fetal pancreatic tissue with even lower levels of PBMC reconstitution [9]. In this study, we achieved human CD45+ engraftment with a median of 11.4 % in spleen and 8.2 % in peripheral blood, which is sufficient to cause allograft rejection.
Generally, the microcapsules act as a barrier in encapsulated islets, and prevent the entry of immune cells, thereby ruling out the possibility of direct cell-mediated allograft rejection. Therefore, any graft rejection might be attributed to immune cells secreting cytokines/chemokines that can enter the capsule through its pores [13]. The cytokines/chemokines may also cause fibrotic overgrowth around the microcapsules to interfere with entry of nutrients, including oxygen [14]. Adult β-cells are particularly sensitive to the adverse effects of proinflammatory cytokines IL-1β, TNF-α, and IFN-γ [15]. Allografted encapsulated islets, but not empty capsules, attract activated macrophages to the surface of the capsules, and such cells release proinflammatory cytokines IL-1β and TNF-α [13]. On the other hand, anti-inflammatory cytokines such as IL-4 and IL-10 protect the pancreatic β-cells against cell death induced by pro-inflammatory cytokines [16]. Therefore, the balance between pro-inflammatory (Th1) and anti-inflammatory (Th2) cytokine levels is crucial in determining the nature of the immune response to induce graft rejection or tolerance.
In our study, we found IL-4 and IL-10 in the peritoneal fluid of hu-NOD/SCID mice. Whereas, the pro-inflammatory cytokines IL-2, IL-12, IL-1β, and TNF-α were undetectable (Table 4). IFN-γ was the only pro-inflammatory cytokine, but only detected at very low levels at day 14. This observation suggests that the immune response is mainly driven by Th2 cells including cytokines (IL-4 and IL-10) involved in graft tolerance [16, 17]. Human islets express IL-4 receptor [18]. Patients with elevated levels of IL-4 have been shown to be resistant to the progression of diabetes [19], and in animal models, IL-4 may also be protective against diabetes development [20, 21]. It is therefore likely that the presence of IL-4 protected the pancreatic β-cells against cell death induced by pro-inflammatory cytokines. Similarly, IL-10 is known to possess strong immunosuppressive properties, and IL-10 upregulation has been shown to enhance islet allograft survival [23, 24]. Thus, the strong immunosuppressive properties of IL-4 and IL-10 might be responsible for the suppressed adverse effect on the encapsulated islets observed in our hu-NOD/SCID mice model. These results raised the possibility that coating the surface of the microcapsules with anti-inflammatory cytokines, or stimulating their production in the diabetic recipient, might be beneficial to the survival of encapsulated human islets transplanted into humans.
In a recent clinical study by Huurman et al., it has been shown that diabetic recipients, whose levels of IL-10 increased after transplantation with human islets, became insulin-independent. Whereas, recipients with non-increased IL-10 levels remained insulin-dependent [25]. The study by Huurman et al. suggests a possible immunoregulatory role of IL-10 in the islet allograft rejection mechanism. IL-10 is generally considered as an immunosuppressive cytokine and its role in autoimmunity and transplantation settings are well documented [26]. The immunosuppressive property of IL-10 is attributed to its direct inhibitory effect on IL-2 and TNF-α production by CD4+ T cells, the downregulation of costimulatory molecules, and the production of pro-inflammatory cytokines [26]. In our study, the levels of IL-2 and TNF-α were undetectable in the humanized mice at all time points possibly due to the immunoregulatory role of IL-10, which supported graft survival. Although the humanized mouse does not seem to be a suitable preclinical model to investigate the mechanisms of allograft rejection of encapsulated human islets, our study emphasizes the specific role of immunoregulatory cytokines for graft survival and prevention of fibrotic overgrowth of encapsulated islets.
The alloreactivity, fibrotic overgrowth, and par-tial reduction of islet viability we observed in this study might in part be attributed to the chemokines found in the peritoneal cavity. Chemokines are associated with β-cell destruction, and their blockade has been shown to prolong islet allograft survival [27-29]. It has also been demonstrated that islets with low levels of MCP-1 work better than those with higher levels of this chemokine [30, 31]. In this study, we detected significantly higher levels of chemokines MCP-1, eotaxin, and IP-10 in the humanized mice compared with non-humanized controls (Table 4). However, the levels of IP-10 were significantly higher than those of MCP-1 and eotaxin on day 14, and this induction might be mediated by IFN-γ [32]. Thus, the partial reduction in islet viability could be attributed to the cytotoxic effects of the chemokines MCP-1, eo-taxin, and IP-10.
In summary, our results show that the outcome of transplanting encapsulated human islets into hu-NOD/SCID mice is very different from that in humans. It showed that the hu-NOD/SCID mouse is not a suitable preclinical animal model to study the fate of encapsulated human islets that are al-lografted, since islets are largely protected from fibrotic overgrowth. This may be because of the anti-inflammatory effects of the cytokines IL-4 and IL-10 secreted in the hu-NOD/SCID mouse. The significance of our results is that it should accelerate efforts needed to develop a suitable animal model that mimics the clinical situation, when encapsulated human islets are allografted into people with type 1 diabetes.
Disclosures (conflict of interests statement): The authors report no conflict of interests.
Financial acknowledgement: The lead author VV, received a scholarship from the Australian Foundation for Diabetes Research.
Author contributions: VV contributed by designing and performing the study, collecting and analyzing data, and writing the paper. JO contributed with human islets, and data analysis. GJG performed data analysis. BET contributed by designing the study, data analysis, and paper writing.
Acknowledgments:
We wish to thank the University of Illinois Foundation, which facilitates the Chicago Diabetes Project, and the Australian Foundation for Diabetes Research, for supplying human islets, and for funding the project. We are grateful to Prof. Julie Kerr-Conte, Assistant Professor Barbara Barbaro, and Professor David Hunkeler for critical reading of the manuscript.
References
- Calafiore R, Basta G, Luca G, Lemmi A, Montanucci MP, Calabrese G, Racanicchi L, Mancuso F, Brunetti P. Microencapsulated pancreatic islet allografts into nonimmunosuppressed patients with type 1 diabetes: first two cases. Diabetes Care 2006. 29(1):137-138. [DOD] [CrossRef]
- Tuch BE, Keogh GW, Williams LJ, Wu W, Foster JL, Vaithilingam V, Philips R. Safety and viability of microencapsulated human islets transplanted into diabetic humans. Diabetes Care 2009. 32(10):1887-1889. [DOD] [CrossRef]
- de Groot M, Schuurs TA, van Schilfgaarde R. Causes of limited survival of microencapsulated pancreatic islet grafts. J Surg Res 2004. 121(1):141-150. [DOD] [CrossRef]
- Vaithilingam V, Sundaram G, Tuch BE. Islet cell transplantation. Curr Opin Organ Transplant 2008. 13(6):633-638. [DOD] [CrossRef]
- Banuelos SJ, Shultz LD, Greiner DL, Burzenski LM, Gott B, Lyons BL, Rossini AA, Appel MC. Rejection of human islets and human HLA-A2.1 transgenic mouse islets by alloreactive human lymphocytes in immunodeficient NOD-scid and NOD-Rag1(null)Prf1(null) mice. Clin Immunol 2004. 112(3):273-283. [DOD] [CrossRef]
- King M, Pearson T, Shultz LD, Leif J, Bottino R, Trucco M, Atkinson MA, Wasserfall C, Herold KC, Woodland RT, et al. A new Hu-PBL model for the study of human islet alloreactivity based on NOD-scid mice bearing a targeted mutation in the IL-2 receptor gamma chain gene. Clin Immunol 2008. 126(3):303-314. [DOD] [CrossRef]
- Gregori S, Mangia P, Bacchetta R, Tresoldi E, Kolbinger F, Traversari C, Carballido JM, de Vries JE, Korthäuer U, Roncarolo MG. An anti-CD45RO/RB monoclonal antibody modulates T cell responses via induction of apoptosis and generation of regulatory T cells. J Exp Med 2005. 201(8):1293-1305. [DOD] [CrossRef]
- Vlad G, D'Agati VD, Zhang QY, Liu Z, Ho EK, Mohanakumar T, Hardy MA, Cortesini R, Suciu-Foca N. Immunoglobulin-like transcript 3-Fc suppresses T-cell responses to allogeneic human islet transplants in hu-NOD/SCID mice. Diabetes 2008. 57(7):1878-1886. [DOD] [CrossRef]
- Brands K, Colvin E, Williams LJ, Wang R, Lock RB, Tuch BE. Reduced immunogenicity of first-trimester human fetal pancreas. Diabetes 2008. 57(3):627-634. [DOD] [CrossRef]
- Rice AM, Wood JA, Milross CG, Collins CJ, McCarthy NF, Vowels MR. Conditions that enable human hematopoietic stem cell engraftment in all NOD-SCID mice. Transplantation 2000. 69(5):927-935. [DOD] [CrossRef]
- Qi M, Strand BL, Mørch Y, Lacik I, Wang Y, Salehi P, Barbaro B, Gangemi A, Kuechle J, Romagnoli T, et al. Encapsulation of human islets in novel inhomogeneous alginate-Ca2+/Ba2+ microbeads: in vitro and in vivo function. Artif Cells Blood Substit Immobil 2008. 36(5):403-420. [DOD] [CrossRef]
- Foster JL, Williams G, Williams LJ, Tuch BE. Differentiation of transplanted microencapsulated fetal pancreatic cells. Transplantation 2007. 83(11):1440-1448. [DOD] [CrossRef]
- de Vos P, Smedema I, van Goor H, Moes H, van Zanten J, Netters S, de Leij LF, de Haan A, de Haan BJ. Association between macrophage activation and function of microencapsulated rat islets. Diabetologia 2003. 46(5):666-673. [DOD]
- de Vos P, Straaten JF, Nieuwenhuizenn AG, de Groot M, Ploeg RJ, De Haan BJ, Van Schilfgaarde R. Why do microencapsulated islet grafts fail in the absence of fibrotic overgrowth? Diabetes 1999. 48(7):1381-1388. [DOD]
- Rabinovitch A, Sumoski W, Rajotte R, Warnock GL. Cytotoxic effects of cytokines on human pancreatic islet cells in monolayer culture. J Clin Endocrinol Metab 1990. 71(1):152-156. [DOD] [CrossRef]
- Souza KL, Gurgul-Convey E, Elsner M, Lenzen S. Interaction between pro-inflammatory and anti-inflammatory cytokines in insulin-producing cells. J Endocrinol 2008. 197(1):139-150. [DOD] [CrossRef]
- Lowry RP. The relationship of IL-4, IL-10, and other cytokines to transplantation tolerance. Transplant Sci 1993. 3:104-112. [DOD]
- Kaminski A, Welters HJ, Kaminski ER, Morgan NG. Human and rodent pancreatic beta-cells express IL-4 receptors and IL-4 protects against beta-cell apoptosis by activation of the PI3K and JAK/STAT pathways. Biosci Rep 2009. 30(3):169-175. [DOD] [CrossRef]
- Wilson SB, Kent SC, Patton KT, Orban T, Jackson RA, Exley M, Porcelli S, Schatz DA, Atkinson MA, Balk SP, et al. Extreme Th1 bias of invariant Valpha24JalphaQ T cells in type 1 diabetes. Nature 1998. 391(6663):177-181. [DOD]
- Rapoport MJ, Jaramillo A, Zipris D, Lazarus AH, Serreze DV, Leiter EH, Cyopick P, Danska JS, Delovitch TL. Interleukin 4 reverses T cell proliferative unresponsiveness and prevents the onset of diabetes in nonobese diabetic mice. J Exp Med 1993. 178(1):87-99. [DOD] [CrossRef]
- Cameron MJ, Arreaza GA, Waldhauser L, Gauldie J, Delovitch TL. Immunotherapy of spontaneous type 1 diabetes in nonobese diabeticmice by systemic interleukin-4 treatment employing adenovirus vector-mediated gene transfer. Gene Ther 2000. 7(21):1840-1846. [DOD] [CrossRef]
- Kim YH, Lim DG, Wee YM, Kim JH, Yun CO, Choi MY, Park YH, Kim SC, Han DJ. Viral IL-10 gene transfer prolongs rat islet allograft survival. Cell Transplant 2008. 17(6):609-618. [DOD] [CrossRef]
- Deng S, Yang ZD, Ketchum RJ, Kucher T, Weber M, Shaked A, Naji A, Brayman KL. Transfer of genes for IL-10 and TGF-bet to isolated human pancreatic islets. Transplant Proc 1997. 29(4):2206. [DOD] [CrossRef]
- Benhamou PY, Mullen Y, Shaked A, Bahmiller D, Csete ME. Decreased alloreactivity to human islets secreting recombinant viral interleukin 10. Transplantation 1996. 62(9):1306-1312. [DOD] [CrossRef]
- Huurman VA, Velthuis JH, Hilbrands R, Tree TI, Gillard P, van der Meer-Prins PM, Duinkerken G, Pinkse GG, Keymeulen B, et al. Allograft-specific cytokine profiles associate with clinical outcome after islet cell transplantation. Am J Transplant 2009. 9(2):382-388. [DOD] [CrossRef]
- Pestka S, Krause CD, Sarkar D, Walter MR, Shi Y, Fisher PB. Interleukin-10 and related cytokines and receptors. Annu Rev Immunol 2004. 22:929-979. [DOD] [CrossRef]
- Baker MS, Chen X, Rotramel AR, Nelson JJ, Lu B, Gerard C, Kanwar Y, Kaufman DB. Genetic deletion of chemokine receptor CXCR3 or antibody blockade of its ligand IP-10 modulates posttransplantation graft-site lymphocytic infiltrates and prolongs functional graft survival in pancreatic islet allograft recipients. Surgery 2003. 134(2):126-133. [DOD] [CrossRef]
- Lee I, Wang L, Wells AD, Ye Q, Han R, Dorf ME, Kuziel WA, Rollins BJ, Chen L, Hancock WW. Blocking the monocyte chemoattractant protein-1/CCR2 chemokine pathway induces permanent survival of islet allografts through a programmed death-1 ligand-1–dependent mechanism. J Immunol 2003. 171(12):6929-6935. [DOD]
- Abdi R, Means TK, Luster AD. Chemokines in islet allograft rejection. Diabetes Metab Res Rev 2003. 19(3):186-190. [DOD] [CrossRef]
- Piemonti L, Leone BE, Nano R, Saccani A, Monti P, Maffi P, Bianchi G, Sica A, Peri G, Melzi R, et al. Human pancreatic islets produce and secrete MCP-1/CCL2: relevance in human islet transplantation. Diabetes 2002. 51(1):55-65. [DOD] [CrossRef]
- Schroppel B, Zhang N, Chen P, Chen D, Bromberg JS, Murphy B. Role of donor-derived monocyte chemoattractant protein-1 in murine islet transplantation. J Am Soc Nephrol 2005. 16(2):444-451. [DOD] [CrossRef]
- Cardozo AK, Proost P, Gysemans C, Chen MC, Mathieu C, Eizirik DL. IL-1beta and IFN-gamma induce the expression of diverse chemokines and IL-15 in human and rat pancreatic islet cells, and in islets from pre-diabetic NOD mice. Diabetologia 2003. 46(2):255-266. [DOD]
This article has been cited by other articles:
|
Beneficial effects of coating alginate microcapsules with macromolecular heparin conjugates-in vitro and in vivo study
Vaithilingam V, Kollarikova G, Qi M, Larsson R, Lacik I, Formo K, Marchese E, Oberholzer J, Guillemin GJ, Tuch BE
Tissue Eng Part A 2014. 20(1-2):324-334
|
|
|
Characterisation of the xenogeneic immune response to microencapsulated fetal pig islet-like cell clusters transplanted into immunocompetent C57BL/6 mice
Vaithilingam V, Fung C, Ratnapala S, Foster J, Vaghjiani V, Manuelpillai U, Tuch BE
Plos One 2013. 8(3):e59120
|
|
|
Transplantation of Pancreatic Islets Immobilized in Alginate-Based Microcapsules: From Animal Studies to Clinical Trials
Qi M, McGarrigle JJ, Wang Y, Marchese E, Bochenek MA, Vaca P, Davis ME, Ahn SJ, Schwartz A, Strand B, Lacik I, Oberholzer J
Micro Nanosyst 2013. 5(3):186-193
|
|
|
Microencapsulation for Human Islet Transplantation
Tuch BE, Vaithilingam V
Micro Nanosyst 2013. 5(3):162-167
|
|
|
Research on the establishment of Balb/c nu/nu mouse diabete model and its subcutaneous islet xenotransplantation
Fu HX, Li H, Jiang L, et al.
J Hepatopancreatobil Surg 2013. 25(3):225-228
|
|
|
Encapsulated cell grafts to treat cellular deficiencies and dysfunction
Krishnamurthy NV, Gimi B
Crit Rev Biomed Eng 2011. 39(6):473-491
|
|
|
Islet transplantation and encapsulation: an update on recent developments
Vaithilingam V, Tuch BE
Rev Diabet Stud 2011. 8(1):51-67
|
|
|
The new generation of beta-cells: replication, stem cell differentiation, and the role of small molecules
Borowiak M
Rev Diabet Stud 2010. 7(2):93-104
|
|
|