Review
Rev Diabet Stud,
2012,
9(1):23-35 |
DOI 10.1900/RDS.2012.9.23 |
The Diabetic Lung - A New Target Organ?
Dario Pitocco1,2, Leonello Fuso2,3, Emanuele G. Conte3, Francesco Zaccardi1, Carola Condoluci3, Giuseppe Scavone1, Raffaele Antonelli Incalzi4, Giovanni Ghirlanda1
1Diabetes Care Unit, Catholic University of Rome, Italy
2Equally contributed to the paper
3Respiratory Disease Unit, Catholic University of Rome, Italy
4Geriatric Medicine, University Campus Bio-Medico, Rome, Italy
Address correspondence to: Dario Pitocco, MD, Servizio di Diabetologia, Policlinico A. Gemelli, L.go A. Gemelli 1, 00168 Roma, Italy, e-mail: dario.pitocco@rm.unicatt.it
Manuscript submitted April 11, 2012; resubmitted April 25, 2012; accepted May 4, 2012.
Keywords: diabetic lung, diabetes, hyperglycemia, respiratory dysfunction, microvascular reserve, ventilatory pump
Abstract
Several abnormalities of the respiratory function have been reported in patients with type 1 and type 2 diabetes. These abnormalities concern lung volume, pulmonary diffusing capacity, control of ventilation, bronchomotor tone, and neuroadrenergic bronchial innervation. Many hypotheses have emerged, and characteristic histological changes have been described in the "diabetic lung", which could explain this abnormal respiratory function. Given the specific abnormalities in diabetic patients, the lung could thus be considered as a target organ in diabetes. Although the practical implications of these functional changes are mild, the presence of an associated acute or chronic pulmonary and/or cardiac disease could determine severe respiratory derangements in diabetic patients. Another clinical consequence of the pulmonary involvement in diabetes is the accelerated decline in respiratory function. The rate of decline in respiratory function in diabetics has been found to be two-to-three times faster than in normal non-smoking subjects, as reported in longitudinal studies. This finding, together with the presence of anatomical and biological changes similar to those described in the aging lung, indicates that the "diabetic lung" could even be considered a model of accelerated aging. This review describes and analyses the current insight into the relationship of diabetes and lung disease, and suggests intensifying research into the lung as a possible target organ in diabetes.
Abbreviations: 99mTc-DTPA - technetium-99m diethylene triamine penta-acetic acid aerosol; ARIC - Atherosclerosis Risk in Communities; BMI - body mass index; CO - carbon monoxide; COPD - chronic obstructive pulmonary disease; DLCO - pulmonary diffusing capacity for carbon monoxide; DLNO - pulmonary diffusing capacity for nitric oxide; FEV1 - forced expiratory volume in one second; FVC - forced vital capacity; HbA1c - glycosylated hemoglobin levels; HRV - heart rate variability; MIBG - metaiodobenzylguanidine; NHANES III - Third National Health And Nutrition Ex-amination Survey; OSA - obstructive sleep apnoea; T1D - type 1 diabetes; T2D - type 2 diabetes; TNF-alpha - tumor necrose factor alpha; TLC - total lung capacity; VO2-peak - peak of oxygen uptake
Introduction
Diabetes is the most common of all endocrine diseases and the fourth to fifth leading cause of death in developed countries [1]. Retinopathy, neuropathy, nephropathy, and cardiovascular dysfunction are common diabetic complications, and contribute significantly to morbidity and mortality. These complications are basically caused by vascular damage, which has a central role in the pathophysiology of diabetes [2, 3]. Despite the presence of a large capillary network in the lung, pulmonary complications of diabetes are frequently disregarded. This is mainly because the alveolar-capillary system is characterized by a great microvascular reserve, and pulmonary abnormalities are commonly subclinical in diabetic patient [4]. However, the loss of microvascular reserve in the lung may become clinically important, with increased risk of hypoxia, in case of acute or chronic pathological lung conditions, including pneumonia, chronic obstructive pulmonary disease, and asthma, or fluid overload secondary to heart failure [5].
The association between diabetes and impaired lung function has been frequently observed. Various respiratory disorders have been described in patients with either type 1 and type 2 diabetes [4]. However, the potential clinical implications of this interrelationship have not yet been fully elucidated. This drawback suggests that further research into the lung as a possible target organ in diabetes is needed [6]. The following sections summarize the current state of knowledge on the association of diabetes and lung disease. We learn from the impressive arguments that diabetes can have a decisive influence on lung function, and this has led us to think about the "diabetic lung", and to guide more attention to this important topic.
Mechanisms of lung damage
Mechanisms underlying lung damage in diabetes are not fully clear. Conceivably, the nonenzymatic glycosylation of proteins in the lungs and chest wall makes the collagen less susceptible to proteolysis and leads to its accumulation in lung connective tissue [7, 8]. This process is mainly driven by hyperglycemia, and thus it is more pronounced in patients with poor metabolic control [7]. In the course of collagen accumulation in the lung, the increased stiffness of both lung parenchyma and chest wall could explain the restrictive functional defect appearing in lung disease. Whereas, the loss of elastic recoil capacity in the lung leads to a dynamic collapse of small airways during exhalation.
Another potential mechanism in lung disease is related to decreased muscle strength, a phenomenon that is also related to insulin-resistance, suggesting a relationship to diabetes [9]. Consequently, myopathic and/or neuropathic changes affecting respiratory muscles could impair the efficiency of the ventilatory pump and thereby contribute to functional lung defects.
Some studies suggested that diabetes may increase the patients' susceptibility to the adverse pulmonary effects of tobacco smoking [10] and airborne particles [11]. Also, insulin resistance could alter lung volume and mechanical function via mediators such as leptin. Indeed, animal studies have provided evidence for leptin as a stimulant of ventilation, whereas researchers have also proposed an important role for leptin in lung maturation and development [5]. Studies further suggest a significant impact of leptin on specific respiratory diseases, including obstructive sleep apnoea-hypopnoea syndrome, asthma, chronic obstructive pulmonary disease (COPD), and lung cancer [5]. Serum leptin levels are usually elevated in obesity, and associated with small lung volumes. In non-obese subjects, serum leptin levels are inversely related to forced expiratory volume in one second (FEV1) [12, 13].
Another hypothesis points to systemic inflammation as a link between diabetes and lung dysfunction. Abnormal regulation of inflammatory mechanisms could cause exaggerated inflammatory responses in the lung, resulting in impaired lung function. Also, the lung can be the primary location of the activation of inflammatory processes, followed by the development of type 2 diabetes. This hypothesis could explain the observation of diminished lung function several years before diabetes diagnosis [14-17]. Furthermore, subjects with type 2 diabetes and inadequate glycemic control had lower FEV1 and forced vital capacity (FVC) than subjects with adequate control, a difference not explained by usual determinants of lung function. Similarly, patients with inadequate control also had significantly higher levels of inflammation markers (TNF-alpha, ferritin, fibrinogen, and C-reactive protein), suggesting a potential involvement of inflammation in impaired lung function [18], in particular in lung fibrosis. In a prospective cohort study involving about 100,000 women, it was found that subjects with COPD had a statistically significant increased risk of type 2 diabetes, and inflammatory markers have been suggested to be the common link [19]. Thus, both systemic and pulmonary inflammation may be implicated in the complex interplay between respiratory and metabolic dysfunction [20].
Histopathological evidence
In patients with diabetes, there is histopathological evidence of thickened alveolar, epithelial, and pulmonary capillary basal lamina. According to post mortem studies, epithelial and capillary basal laminas of alveoli are significantly thicker in diabetics than in age-matched controls [21]. The degree of thickening did not correlate significantly with patient age or with duration of diabetes, but correlated significantly with the thickness of basal laminas in renal tubules and muscle capillaries [21, 22]. These microvascular abnormalities are frequently associated with histological changes in the lung parenchyma, such as nodular fibrosis [23], and with increased extracellular matrix and connective tissue synthesis in the lung parenchyma of animals [24]. Experimental data obtained in rats with streptozotocin-induced diabetes have shown that hyperglycemia increased the content of collagen and elastin in the lung [24]. In this regard, the volume proportion of alveolar walls was increased at the expense of alveolar air, and air spaces were diminished in size [25]. Changes in collagen metabolism have been related to an apparent accelerated ageing of human collagen in diabetes, probably because one or more properties of collagen could be altered in ways that simulate accelerated ageing [26].
Functional changes in the respiratory tract of diabetes patients and possible pathogenic mechanisms
Several abnormalities in indices of respiratory function have been reported in patients with diabetes. The main functional changes in the respiratory tract are summarized in Table 1.
Table
1.
Main changes in respiratory functional index in diabetes mellitus |
|
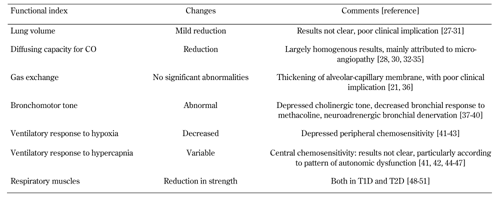 |
 |
Legend:
The table is adapted from: Guazzi M, Fuso L, European respiratory monograph, 2009, p. 217-239. Find references [27-51] in the reference list at the end of the article. Abbreviations: CO - carbon monoxide, T1D - type 1 diabetes, T2D - type 2 diabetes. |
|
Pulmonary diffusing capacity for carbon monoxide (DLCO)
The first demonstration of a deficit in lung function in diabetes was about 35 years ago. Schuyler et al. showed that, in a small number of young patients with type 1 diabetes, the elastic recoil of the lung was reduced compared with healthy controls [52]. The authors attributed this finding to the effects of diabetes on the elastic structures of the lung. The reduction of lung elastic recoil in diabetic patients was later confirmed in another cross-sectional study [32]. It was also shown that the diabetes patients had a reduced pulmonary diffusing capacity for carbon monoxide (DLCO) [32]. This effect was attributed to vascular injury of pulmonary capillaries related to diabetic microangiopathy [30, 33, 34].
The reduction of DLCO in patients with both type 1 and type 2 diabetes has been detected in several studies. Today, there is agreement with this finding. DLCO is a parameter that is easily measured during lung function testing. It is influenced by two main components: alveolar-capillary membrane conductance and pulmonary capillary blood volume. Several studies have shown that diabetes is frequently associated with histopathological alterations acting on both determinants of DLCO. In particular, one of the most frequently described histopathological alterations is the thickening of the pulmonary capillary basal lamina which, together with the alveolar epithelium thickening, contributes to reduced gas diffusion velocity through the alveolar-capillary membrane [21, 36]. Using a sensitive method such as 99mTc-DTPA aerosol scintigraphy, Ozsahin et al. demonstrated a reduction in the permeability of the alveolar-capillary basement membrane in diabetic patients even without any evidence for DLCO reduction [53]. Ljubic et al. showed that the reduced alveolar-capillary permeability could be caused by changes in collagen and elastin [35]. Other authors emphasized the role of non-enzymatic protein glycation in the extracellular matrix as the main cause of alteration in protein turnover [54]. Forgiarini et al. pointed out the role of local oxidative stress in endothelial dysfunction and alteration or thickening of lung interstitium via an increase in collagen protein synthesis and accelerated cross-linking [55]. Therefore, similar as in other organs, diabetes seems to cause injury in the pulmonary microcirculation by increasing vessel wall thickness. In fact, considering its large vascular network and richness in collagen and elastin, the pulmonary system is susceptible to microvascular damage and non-enzymatic glycation in diabetes. The presence of these alterations could be regarded as the manifestation of diabetic microangiopathy. To confirm these considerations, it has been demonstrated that the reduction of DLCO in diabetic patients correlates with the severity of other vascular complications, such as retinopathy and renal microangiopathy [30, 34, 35, 56].
The increased thickness of the alveolar-capillary barrier and the expansion of the interstitium are associated with a reduction of alveolar space and a narrowing of the pulmonary capillary network [21, 36]. Pulmonary capillary blood volume represents the amount of blood in contact with the ventilated alveoli. Any alteration in pulmonary vasculature may lead to redistribution of pulmonary circulation, potentially causing ventilation/perfusion mismatch and impaired gas exchange. Recently, Wheatley et al. have demonstrated that in diabetics the lung fails to recruit pulmonary capillaries in response to increased requests, as during physical exercise or after postural changes [57]. The same authors measured both DLCO and the transfer of nitric oxide (DLNO) in these patients, to distinguish between the two effects, namely the reduced pulmonary capillary blood volume and the reduction of alveolar-capillary membrane conductance. This was done as the high NO conductance in blood makes DLNO an independent measure of alveolar-capillary transfer. The authors found that the reduction of DLCO in diabetic patients is a combination of a decrease in pulmonary capillary blood volume and alveolar-capillary membrane conductance, both expressions of diabetic microangiopathy and lung involvement [58]. In this prospective study, DLCO could be used as a simple and non-invasive test for the assessment of pulmonary vascular damage in diabetic patients, even if this test is not very sensitive.
A method to increase the diagnostic sensitivity of DLCO in the detection of severe diabetic micro-angiopathy is to measure the postural changes in DLCO. It is well known that in normal subjects the increase in DLCO in the supine, compared to the sitting position, is related to the recruitment of the upper lobe pulmonary capillaries, thus increasing the total volume of pulmonary blood volume [59, 60]. Although normal when measured in sitting position, DLCO did not increase with a change from sitting to supine position in patients with type 1 diabetes, compared to healthy subjects [61]. This observation has been attributed to the lack of ability to increase capillary volume in the supine position because of diabetic microangiopathy. Therefore, measurement of DLCO in postural changes could be a useful and a more sensitive tool than simple measurement of DLCO in sitting position in the early diagnosis of diabetic lung complications [61].
Another possible explanation of the DLCO changes described in diabetic patients can also be related to the dysfunction in the autonomic nervous system, which is often reported in type 1 diabetes. Indeed, it was found very recently that reduced DLCO and cardiac autonomic dysfunction are significantly associated. This relationship was determined by the analysis of heart rate variability (HRV), which is a very sensitive method [62].
Lung volumes
There is substantial evidence of pulmonary vascular damage related to diabetic microangiopathy, which is responsible, at least in part, for the reduction in DLCO. However, conflicting data exists regarding the effects of diabetes on respiratory mechanics and lung volume [4]. Initially, it was reported that patients with diabetes had reduced spirometric measures [28]. However, these findings were not confirmed by subsequent studies, which found nearly normal lung volumes in diabetic patients, similar as in control subjects [61]. This led to the rejection of a correlation between lung function and glycemic control or duration of diabetes [30, 31].
On the other hand, Niranjan et al. later found a significant reduction in total lung capacity (TLC) in type 1 diabetic patients compared with healthy subjects. This reduction was more pronounced in the subgroup of patients with poor glycemic control [27]. However, lung volumes were expressed in absolute values instead of percentages of predicted values. Another epidemiological study has reported a modest reduction in lung volumes of type 2 diabetic patients, about 10% lower than the predicted values derived from a normal population [29]. Lung function was also measured in a cohort of 495 patients with type 2 diabetes without any history of pulmonary disease, where 125 of the patients repeated these measurements after a follow-up of approximately 7 years [63]. FVC, FEV1, and vital capacity (VC) values measured at baseline were at the lower limits of the normally predicted values, but 29 of the 125 patients (23% of the patients who took part in the follow-up) had baseline FEV1 < 70% of the predicted value and/or a VC < 80% of the predicted value, without evidence of associated lung disease [63].
The Third National Health And Nutrition Examination Survey (NHANES III 2005) [64] found that previously diagnosed diabetes patients had lower FEV1 than non-diabetics. Also, the Framingham Offspring Cohort and the Heart and Health Study reported a reduced FEV1 in diabetic patients in comparison to non-diabetics [10, 65]. In a cross-sectional analysis, the Atherosclerosis Risk in Communities (ARIC) study has reported that the values of FEV1 and FVC were significantly lower in type 2 diabetes than in healthy controls [66]. Using more sensitive functional tests to evaluate the respiratory mechanics such as the measurement of dynamic compliance, some authors have demonstrated a reduction of this parameter in type 1 diabetes patients, thus indirectly detecting the presence of a peripheral airway obstruction in these patients [67, 68].
A recent meta-analysis pooled the data of 40 studies related to the difference in mean respiratory function between a total of 3182 patients with diabetes and 27,070 controls. The authors found a moderate, but statistically significant, impaired lung function tending towards a restrictive pattern [69]. In sub-analyses, this association seemed to be more pronounced for type 2 diabetes. Although not clinically relevant under stable conditions, this impairment means a loss of pulmonary reserves which could eventually become problematic in case of increased pulmonary demands such as repeated respiratory infections.
The pathogenic mechanisms leading to a reduced lung function in diabetic patients have not been fully revealed. It has been suggested that the glycosylation of proteins such as collagen in the bronchial tree can explain the deficit in lung function, especially in diabetic patients with poor control of blood glucose [7]. Data on anatomic lung abnormalities reported in patients with diabetes can, at least in part, explain the pulmonary functional deficit. In addition to the alterations in the pulmonary vascular system and the alveolar-capillary membrane, parenchymal damages to lungs of diabetes patients has also been reported [8, 23-25]. Farina et al. demonstrated the presence of areas of lung fibrosis with specific nodular fibrosis pattern [23]. To support the hypothesis of a link between diabetes and lung fibrosis, a case-control study, estimating the odds ratio of diabetes as a risk factor for idiopathic fibrosis, found a correlation between these two diseases [70].
It has also been suggested that diabetes patients are more susceptible to the adverse effects caused by tobacco smoke and environmental pollutants [10, 11]. Another attractive hypothesis is that the systemic inflammation could be the link between diabetes and lung. In diabetic patients, systemic inflammation is associated with endothelial dysfunction [71], and may contribute to the development of diseases of the air passages, as in asthma and chronic obstructive pulmonary disease. In turn, systemic inflammation in chronic lung diseases may be responsible for the development of comorbidities such as diabetes [15] and insulin resistance [72]. Therefore, it is conceivable that inflammatory markers of reduced lung function in non-diabetic subjects, such as fibrinogen [73], are also associated with the development of diabetes [74].
Glycemic control appears to play a key role in the association of reduced lung function and diabetes. This was evidenced by a cross-sectional study based on the Framingham Offspring Cohort which found significant correlations between diabetes, fasting plasma glucose levels, and lung function in approximately 3,200 subjects [10]. However, there is disagreement on this hypothesis when the results of other longitudinal studies are considered. In two studies, the association between glycosylated hemoglobin levels (HbA1c) and spirometric measurements was weak or not present [29, 75]. In another cross-sectional population study, a negative association between plasma glucose level and FVC and/or FEV1 was found [76]. Basically, it should be noted that a good glycemic control and regular administration of insulin could positively influence functional lung parameters. In fact, it has been shown that insulin infusion results in an acute improvement in alveolar-capillary membrane conductance, suggesting a beneficial effect on lung diffusing capacity and gas exchange independent of its metabolic effects [77].
Bronchomotor tone and control of ventilation
Several authors have reported disorders in bronchomotor tone and control of ventilation among diabetic patients with autonomic neuropathy, a complication which may affect up to 30% of the diabetic population, and which is associated with increased morbidity and mortality [37]. Noradrenergic dysfunction in bronchial innervation has been demonstrated in a group of patients with type 1 diabetes who underwent lung ventilation scintigraphy with 123-I-metaiodobenzylguanidine (MIBG) [40]. The radiotracer was taken up in the lung by the same mechanism that controls the uptake of norepinephrine and selectively binds bronchial neuroadrenergic receptors [78]. Diabetic patients with autonomic neuropathy showed an increased lung uptake of 123-MIBG and a decreased rate of its clearance [40]. This result pointed to a dysfunction in bronchial neuroadrenergic innervation; the magnitude of which was directly related to the severity of the autonomic defect. Autonomic neuropathy can cause functional impairment of the respiratory system through damage to the bronchial neuroadrenergic innervation, thus altering the ventilatory response to central and peripheral stimuli.
In particular, Bertherat et al. have reported about a reduced bronchoconstrictive response induced by methacholine in 22 insulin-dependent diabetes patients with autonomic neuropathy [38, 39]. In another study, bronchial responsiveness to methacholine was significantly correlated with several indices of cardiovascular autonomic control in patients with type 1 diabetes [39]. Fonseca et al. gave a possible explanation of these findings. They showed that the reduction of the parasympathetic bronchomotor tone may cause an increase in airway caliber at baseline in diabetic patients with autonomic neuropathy compared to those without autonomic disorders [79].
Non-univocal data are reported in the literature relating to the influence of diabetic dysautonomia on the control of ventilation. It has been shown that diabetes is associated with periodic breathing, a respiratory disorder induced by abnormal central respiratory control. However, even if there is a substantial homogeneity of findings regarding a depressed chemosensibility to hypoxia in diabetic patients with autonomic neuropathy [41, 42], conflicting data have been reported about the chemosensibility to hypercapnia. In fact, the ventilatory response to hypercapnia was found increased, normal, or reduced in apparently comparable populations of diabetic patients with autonomic neuropathy [42, 44, 45]. It is possible that the differences in the results regarding the response to hypercapnia are caused by different degrees of severity of dysautonomia, different patterns of the autonomic nervous system disturbance, or different stimulus intensity [47]. Indeed, Tantucci et al. demonstrated that the response to hypercapnia is reduced in diabetics with parasympathetic dysautonomia, while hypercapnic drive was slightly increased in patients with both sympathetic and parasympathetic damage [46]. These findings suggest that the sympathetic component seems to play an important role in modulating the response of the respiratory centers to hypercapnic stimulation [46]. It also shows that different patterns of autonomic dysfunction in diabetes could influence the chemosensibility in various directions. Finally, at least a part of the ventilatory response to hypercapnia is dependent on peripheral chemoreceptors of carotid glomus [80], which may contribute to the heterogeneity of results reported in the literature.
In partial contrast with these studies, it has recently been shown that patients with type 1 diabetes have a reduced ventilatory response to hypercapnia independent from the presence of autonomic neuropathy [81]. This finding suggests that diabetes per se, and not dysautonomia, is the main cause of depressed hypercapnic chemosensibility. The same study has also demonstrated a significant correlation between the reduction in ventilatory response to hypercapnia and DLCO, which could be used to identify diabetic patients with abnormal respiratory response to hypercapnia [81]. The latter finding seems to suggest that an alteration of cerebral microcirculation may contribute, at least in part, to reduced hypercapnic chemosensibility in diabetic patients as the cerebral vascular reactivity to carbon dioxide partly mediates the ventilatory effects of carbon dioxide on the central chemoceptors. Together, these data provide support for the hypothesis that DLCO is a promising index of the severity of abnormal respiratory response in diabetes.
Respiratory muscle function testing
Few studies have investigated the respiratory muscle function in diabetes, mainly in patients with type 1 diabetes. In these studies, the levels of respiratory muscle strength have been found to be generally low [48-50]. A recent study with type 2 diabetic patients showed a modest reduction in respiratory muscle strength, inversely correlated to the degree of metabolic control, expressed by the HbA1c level [51]. In the same study, the endurance of the respiratory muscles was reduced in more than one third of patients, and this deficit was prevalent in patients with diabetic microvascular complications. These data may suggest that the respiratory muscular deficit in strength or endurance could have different related patterns of anatomical and/or physiological alteration [51]. Regardless of the pathogenetic mechanisms involved, any deficit of respiratory muscle function may in turn lead to lower lung volumes, thus contributing, at least in part, to the restrictive functional impairment that is often seen in these patients.
Cardiopulmonary exercise test
Various authors have demonstrated that, during the incremental exercise test, type 1 diabetic patients have a peak of oxygen uptake (VO2-peak), which is lower than in healthy controls [82, 83]. The VO2-peak indicates the maximum amount of oxygen that can be used by an individual during a physical activity involving large muscle groups. The peak gradually increases in intensity until exhaustion. It is an indicator of the maximum aerobic power, usually measured during a cardiopulmonary incremental exercise test, which evaluates the combined cardiovascular, respiratory, muscular, and neurological responses to physical exercise. The main factor reducing the peak exercise parameters in type 1 diabetic patients seems to be glycemic control, since VO2-peak, workload, heart rate, and cardiac output during exercise were worse in diabetic patients with elevated serum levels of HbA1c [84].
Komatsu et al. observed that individuals with type 1 diabetes were characterized by the same aerobic capacity as non-diabetic subjects if they were in training, although the anaerobic threshold was lower than non-diabetic athletes [85]. This could suggest that highly trained individuals with diabetes and good glycemic control can achieve the same cardiopulmonary exercise response as trained subjects without diabetes. Regarding the exercise capacity in type 2 diabetic patients, the same detrimental effects of diabetes on VO2 kinetics, VO2 peak, and ventilatory threshold have been described [86-89]. However, the results of studies evaluating whether glycemic control could affect exercise tolerance in patients with type 2 diabetes are conflicting [86, 87, 90-92].
Overall, these findings emphasize the role of hyperglycemia in oxygen transport and utilization. In these studies, poor glycemic control seemed to have a stronger detrimental effect on chronotropic heart response during sub-maximal exercise, and on cardiovascular response to exercise, than on pulmonary response [93]. Nevertheless, the histopathological changes in diabetic lung parenchyma, interstitium, and vasculature may be responsible for a small functional reserve of the lung which, generally sub-clinical, could become evident in situations of increased functional request such as during physical exercise. Also, the alteration of the ventilatory response related to diabetic dysautonomia may explain a reduced tolerance to exercise in these patients.
Decline in lung function over time and its clinical implication
A significant time-related effect of lung injury caused by diabetes has been detected in several longitudinal studies, showing an accelerated decline in lung function in patients with diabetes. However, data are not completely in agreement on this topic, as two other longitudinal studies, based on follow-up periods of 5 and 15 years, respectively, have reported a similar decline in FVC and FEV1 in diabetic and in non-diabetic subjects [94, 95]. However, these studies had some limitations. In the first one, 126 subjects who developed diabetes during the follow-up showed a decline in lung function that was at least twice that of non-diabetics [94]. The second study has limitations in the definition of diabetes, since the diagnosis was either self-reported by the patients or based on a single measurement of fasting blood glucose [95]. Subsequent studies have clearly demonstrated an accelerated decline in lung function in patients with diabetes. Davis et al. followed 125 patients with type 2 diabetes for 7 years, and found a decline of 71 ml/year in FEV1 [63], which is more than twice that expected in healthy subjects [96]. This accelerated decrease in lung function was related to poor glycemic control and independent of cigarette smoking. In the same study, a multivariate analysis of the recorded data showed that the FEV1 decline was the only variable significantly associated with the overall mortality in these patients [63]. This evidence suggests that lung dysfunction in diabetes qualifies as an important marker of increased risk of mortality. A periodic monitoring of spirometric indices could be regarded as an important prognostic indicator [97].
A recent study in lung function in a smaller group of type 1 diabetic patients followed up for a period of 5 years showed similar results regarding the time-related decline [98]. In these patients, a decline of 68 ml/year in FEV1 was observed, which was very similar to the results obtained in the previous study. The FEV1 decline, unrelated to the quality of glycemic control, was almost equal in the subgroups of patients with or without autonomic neuropathy. Also, it affected other functional parameters, such as DLCO and neuroadrenergic bronchial innervation, measured by lung ventilation scintigraphy with MIBG [98]. Table 2 shows an overview of the major longitudinal studies which found an accelerated decline in lung function in diabetic patients. Overall, the abovementioned studies showed that lung complications are a manifestation of a long-lasting hyperglycemia, similar as other diabetes complications such as nephropathy and retinopathy.
Table
2.
Major longitudinal studies demonstrating an accelerated decline in lung function in diabetes |
|
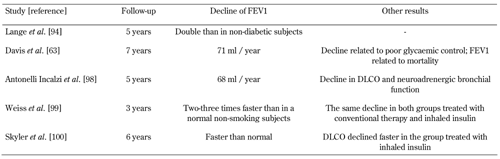 |
 |
Legend:
Find references [63, 94, 98-100] in the reference list at the end of the article. Abbreviations: DLCO - pulmonary diffusing capacity for carbon monoxide, FEV1 - forced expiratory volume in one second. |
|
Potential effects of inhaled insulin on the respiratory tract
It is known that inhaled insulin can be used to achieve better metabolic control in diabetes and increased compliance and adherence to treatment by the patient [101]. However, some questions need to be answered regarding this treatment option. The first is whether impaired lung function could result in a reduced effect of such a therapy. It can be assumed that the microangiopathy and the reduced diffusing capacity of the alveolar-capillary membrane may have adverse effects on the efficacy of inhalation therapy [102]. The second issue is whether inhaled insulin could contribute to lung injury, and thus increase the accelerated decline in lung function in diabetic patients. To date, these questions have not been answered adequately. Some recently published clinical trials have demonstrated greater efficacy of inhaled insulin in achieving glycemic control than traditional therapy in both type 1 and type 2 diabetes, without significant differences in major functional lung indices between the group treated with inhaled insulin and that treated with subcutaneous administration of insulin [99, 100, 103, 104]. However, the follow-up in these studies was between 3 and 6 months, probably too short to detect possible differences between the groups. Other possible limitations of the studies are the following:
- The use of FEV1 alone as the main functional index, which may not fully reflect changes in caliber of the distal airways.
- The lack of measures on bronchial hyper-responsiveness to inhaled insulin.
- The absence of data, even in animal models, which exclude possible negative effects on the bronchial and parenchymal lung anatomy.
- The involvement of highly selected patients, which may limit the extension of the results obtained in the general population [105].
These considerations do not support the use of inhaled insulin in the treatment of diabetes.
Diabetes and obstructive sleep apnoea
Subjects with obstructive sleep apnoea (OSA) are more likely to have diabetes than those without OSA [106]. Moreover, OSA was shown to be independently associated with other neuroendocrine metabolic changes that might favor the development of type 2 diabetes [107]. In particular, OSA is known to affect glucose metabolism, insulin sensitivity, pancreatic beta-cell function, and night sleep quality, resulting in daytime fatigue and sleepiness [108]. These effects can contribute to physical inactivity, which may predispose affected persons, directly and indirectly, to develop obesity and diabetes.
Besides increasing the risk of type 2 diabetes, OSA may itself be affected by diabetes. A study found that 23% of the diabetes population had OSA. After correction for BMI, which explained 13% of the variance in OSA, diabetes explained a further 8% [109]. Until recently, the prevailing opinion was that the conditions of OSA and diabetes are linked by obesity. However, recent reports suggest that they may also be independently associated. Consequently, the incidence of one is higher when the other is present, and both are aggravated when in association with obesity.
Conclusions
Although not fully clarified, the consequences of abnormal lung function in patients with diabetes are clinically relevant. There is evidence that the lung is another target organ of diabetes. Several histopathological features of the lung seem to be related to diabetes [6]. However, the alteration of some spirometric indices does not identify a specific underlying disease, and does not appear to cause clinically significant alterations in these patients at rest. Also, pulmonary function tests such as spirometry performed routinely can be normal in diabetes patients, and do not need to reveal functional deficits which can only be revealed with more sensitive tests.
Regarding diabetic microangiopathy, although a clinically significant consequence in pulmonary gas exchange resulting in respiratory failure has not been reported, the reduction in DLCO reflects the interplay of vascular and parenchymal factors. The sensitivity could be increased both in sitting and supine position when evaluating the DLCO parameter.
Abnormal regulation of bronchomotor tone and neuroadrenergic dysfunction is widespread in patients with autonomic diabetes-related neuropathy. It is possible that these changes negatively affect the prognosis of respiratory infections which are more frequent and more severe in these patients. It is also conceivable that the abovementioned functional deficits can depress the host defense of the airways and the mucociliary clearance, thereby helping to promote the development of lower respiratory tract infections and/or to delay healing. Finally, the derangements of the autonomic innervation with consequent reduction of both peripheral and central chemosensibility may be involved in the altered perception of breathlessness and in anomalous patterns in the ventilatory response to exercise, as frequently observed in diabetic patients with dysautonomia [26, 59]. Therefore, periodic monitoring of the respiratory function in patients with diabetes is an appropriate action, to measure the progression of systemic microangiopathy, and could have prognostic significance since the reduction in some functional indices has been shown to correlate with mortality. On the other hand, if the respiratory function is normal, then it is necessary to preserve this condition of normality by identifying, controlling, or eliminating all risk factors that may worsen lung function such as cigarette smoking, poor glycemic control, and cardiopulmonary diseases.
Disclosure: The authors report no conflict of interests.
References
- King H, Aubert RE, Herman WH. Global burden of diabetes, 1995-2025: prevalence, numerical estimates, and projections. Diabetes Care 1998. 21(9):1414-1431. [DOD] [CrossRef]
- Bowden DW, Cox AJ, Freedman BI, Hugenschimdt CE, Wagenknecht LE, Herrington D, Agarwal S, Register TC, Maldjian JA, Ng MC, et al. Review of the Diabetes Heart Study (DHS) family of studies: a comprehensively examined sample for genetic and epidemiological studies of type 2 diabetes and its complications. Rev Diabet Stud 2010. 7(3):188-201. [DOD] [CrossRef]
- Murea M, Ma L, Freedman BI. Genetic and environmental factors associated with type 2 diabetes and diabetic vascular complications. Rev Diabet Stud 2012. 9(1):6-22. [DOD] [CrossRef]
- Goldman MD. Lung dysfunction in diabetes. Diabetes Care 2003. 26(6):1915-1918. [DOD] [CrossRef]
- Hsia CC, Raskin P. Lung function changes related to diabetes mellitus. Diabetes Technol Ther 2007. 9(Suppl 1):S73-S82. [DOD]
- Sandler M. Is the lung a 'target organ' in diabetes mellitus? Arch Intern Med 1990. 150(7):1385-1388. [DOD]
- Cavan DA, Parkes A, O'Donnell MJ, Freeman W, Cayton RM. Lung function and diabetes. Respir Med 1991. 85(3):257-258. [DOD] [CrossRef]
- Ofulue AF, Thurlbeck WM. Experimental diabetes and the lung. II. In vivo connective tissue metabolism. Am Rev Respir Dis 1988. 138(2):284-289. [DOD]
- Lazarus R, Sparrow D, Weiss ST. Handgrip strength and insulin levels: cross-sectional and prospective associations in the Normative Aging Study. Metabolism 1997. 46(11):1266-1269. [DOD] [CrossRef]
- Walter RE, Beiser A, Givelber RJ, O'Connor GT, Gottlieb DJ. Association between glycemic state and lung function: the Framingham Heart Study. Am J Respir Crit Care Med 2003. 167(6):911-916. [DOD] [CrossRef]
- Zanobetti A, Schwartz J. Are diabetics more susceptible to the health effects of airborne particles? Am J Respir Crit Care Med 2001. 164(5):831-833. [DOD]
- O'Donnell CP, Tankersley CG, Polotsky VP, Schwartz AR, Smith PL. Leptin, obesity, and respiratory function. Respir Physiol 2000. 119(2-3):163-170. [DOD] [CrossRef]
- Sin DD, Man SF. Impaired lung function and serum leptin in men and women with normal body weight: a population based study. Thorax 2003. 58(8):695-698. [DOD] [CrossRef]
- Litonjua AA, Lazarus R, Sparrow D, Demolles D, Weiss ST. Lung function in type 2 diabetes: the Normative Aging Study. Respir Med 2005. 99(12):1583-1590. [DOD] [CrossRef]
- Engstrom G, Janzon L. Risk of developing diabetes is inversely related to lung function: a population-based cohort study. Diabet Med 2002. 19(2):167-170. [DOD] [CrossRef]
- Yeh HC, Punjabi NM, Wang NY, Pankow JS, Duncan BB, Brancati FL. Vital capacity as a predictor of incident type 2 diabetes: the Atherosclerosis Risk in Communities study. Diabetes Care 2005. 28(6):1472-1479. [DOD] [CrossRef]
- Ford ES, Mannino DM. Prospective association between lung function and the incidence of diabetes: findings from the National Health and Nutrition Examination Survey Epidemiologic Follow-up Study. Diabetes Care 2004. 27(12):2966-2970. [DOD] [CrossRef]
- Dennis RJ, Maldonado D, Rojas MX, Aschner P, Rondon M, Charry L, Casas A. Inadequate glucose control in type 2 diabetes is associated with impaired lung function and systemic inflammation: a cross-sectional study. BMC Pulm Med 2010. 10:38. [DOD] [CrossRef]
- Rana JS, Mittleman MA, Sheikh J, Hu FB, Manson JE, Colditz GA, Speizer FE, Barr RG, Camargo CA Jr. Chronic obstructive pulmonary disease, asthma, and risk of type 2 diabetes in women. Diabetes Care 2004. 27(10):2478-2484. [DOD] [CrossRef]
- Fabbri LM, Luppi F, Beghe B, Rabe KF. Complex chronic comorbidities of COPD. Eur Respir J 2008. 31(1):204-212. [DOD] [CrossRef]
- Vracko R, Thorning D, Huang TW. Basal lamina of alveolar epithelium and capillaries: quantitative changes with aging and in diabetes mellitus. Am Rev Respir Dis 1979. 120(5):973-983. [DOD]
- Weynand B, Jonckheere A, Frans A, Rahier J. Diabetes mellitus induces a thickening of the pulmonary basal lamina. Respiration 1999. 66(1):14-19. [DOD] [CrossRef]
- Farina J, Furio V, Fernandez-Acenero MJ, Muzas MA. Nodular fibrosis of the lung in diabetes mellitus. Virchows Arch 1995. 427(1):61-63. [DOD]
- Kida K, Utsuyama M, Takizawa T, Thurlbeck WM. Changes in lung morphologic features and elasticity caused by streptozotocin-induced diabetes mellitus in growing rats. Am Rev Respir Dis 1983. 128(1):125-131. [DOD]
- Ofulue AF, Kida K, Thurlbeck WM. Experimental diabetes and the lung. I. Changes in growth, morphometry, and biochemistry. Am Rev Respir Dis 1988. 137(1):162-166. [DOD] [CrossRef]
- Hamlin CR, Kohn RR, Luschin JH. Apparent accelerated aging of human collagen in diabetes mellitus. Diabetes 1975. 24(10):902-904. [DOD] [CrossRef]
- Niranjan V, McBrayer DG, Ramirez LC, Raskin P, Hsia CC. Glycemic control and cardiopulmonary function in patients with insulin-dependent diabetes mellitus. Am J Med 1997. 103(6):504-513. [DOD] [CrossRef]
- Asanuma Y, Fujiya S, Ide H, Agishi Y. Characteristics of pulmonary function in patients with diabetes mellitus. Diabetes Res Clin Pract 1985. 1(2):95-101. [DOD] [CrossRef]
- Davis TM, Knuiman M, Kendall P, Vu H, Davis WA. Reduced pulmonary function and its associations in type 2 diabetes: the Fremantle Diabetes Study. Diabetes Res Clin Pract 2000. 50(2):153-159. [DOD] [CrossRef]
- Mori H, Okubo M, Okamura M, Yamane K, Kado S, Egusa G, Hiramoto T, Hara H, Yamakido M. Abnormalities of pulmonary function in patients with non-insulin-dependent diabetes mellitus. Intern Med 1992. 31(2):189-193. [DOD] [CrossRef]
- Benbassat CA, Stern E, Kramer M, Lebzelter J, Blum I, Fink G. Pulmonary function in patients with diabetes mellitus. Am J Med Sci 2001. 322(3):127-132. [DOD] [CrossRef]
- Sandler M, Bunn AE, Stewart RI. Cross-section study of pulmonary function in patients with insulin-dependent diabetes mellitus. Am Rev Respir Dis 1987. 135(1):223-229. [DOD]
- Strojek K, Ziora D, Sroczynski JW, Oklek K. Pulmonary complications of type 1 (insulin-dependent) diabetic patients. Diabetologia 1992. 35(12):1173-1176. [DOD] [CrossRef]
- Innocenti F, Fabbri A, Anichini R, Tuci S, Pettina G, Vannucci F, De Giorgio LA, Seghieri G. Indications of reduced pulmonary function in type 1 (insulin-dependent) diabetes mellitus. Diabetes Res Clin Pract 1994. 25(3):161-168. [DOD] [CrossRef]
- Ljubic S, Metelko Z, Car N, Roglic G, Drazic Z. Reduction of diffusion capacity for carbon monoxide in diabetic patients. Chest 1998. 114(4):1033-1035. [DOD] [CrossRef]
- Normal function of the pulmonary bed with thickened basal membrane in diabetes mellitus. Am Rev Respir Dis 1988. 137(Suppl 2):272. [DOD]
- Bottini P, Scionti L, Santeusanio F, Casucci G, Tantucci C. Impairment of the respiratory system in diabetic autonomic neuropathy. Diabetes Nutr Metab 2000. 13(3):165-172. [DOD]
- Pieron M, Scheen AJ, Corhay JL, Radermecker MF, Lefebvre PJ. Bronchial reactivity in diabetic patients. Rev Mal Respir 1997. 14(5):379-385. [DOD]
- Bertherat J, Lubetzki J, Lockhart A, Regnard J. Decreased bronchial response to methacholine in IDDM patients with autonomic neuropathy. Diabetes 1991. 40(9):1100-1106. [DOD] [CrossRef]
- Antonelli Incalzi R, Fuso L, Giordano A, Pitocco D, Maiolo C, Calcagni ML, Ghirlanda G. Neuroadrenergic denervation of the lung in type I diabetes mellitus complicated by autonomic neuropathy. Chest 2002. 121(2):443-451. [DOD] [CrossRef]
- Montserrat JM, Cochrane GM, Wolf C, Picado C, Roca J, Agusti Vidal A. Ventilatory control in diabetes mellitus. Eur J Respir Dis 1985. 67(2):112-117. [DOD]
- Nishimura M, Miyamoto K, Suzuki A, Yamamoto H, Tsuji M, Kishi F, Kawakami Y. Ventilatory and heart rate responses to hypoxia and hypercapnia in patients with diabetes mellitus. Thorax 1989. 44(4):251-257. [DOD] [CrossRef]
- Weisbrod CJ, Eastwood PR, O'Driscoll G, Green DJ. Abnormal ventilatory responses to hypoxia in type 2 diabetes. Diabet Med 2005. 22(5):563-568. [DOD] [CrossRef]
- Soler NG, Eagleton LE. Autonomic neuropathy and the ventilatory responses of diabetics to progressive hypoxemia and hypercarbia. Diabetes 1982. 31(7):609-614. [DOD] [CrossRef]
- Wanke T, Abrahamian H, Lahrmann H, Formanek D, Merkle M, Auinger M, Zwick H, Irsigler K. No effect of naloxone on ventilatory response to progressive hypercapnia in IDDM patients. Diabetes 1993. 42(2):282-287. [DOD] [CrossRef]
- Tantucci C, Scionti L, Bottini P, Dottorini ML, Puxeddu E, Casucci G, Sorbini CA. Influence of autonomic neuropathy of different severities on the hypercapnic drive to breathing in diabetic patients. Chest 1997. 112(1):145-153. [DOD] [CrossRef]
- Tantucci C, Bottini P, Fiorani C, Dottorini ML, Santeusanio F, Provinciali L, Sorbini CA, Casucci G. Cerebrovascular reactivity and hypercapnic respiratory drive in diabetic autonomic neuropathy. J Appl Physiol 2001. 90(3):889-896. [DOD]
- Heimer D, Brami J, Lieberman D, Bark H. Respiratory muscle performance in patients with type 1 diabetes. Diabet Med 1990. 7(5):434-437. [DOD] [CrossRef]
- Scano G, Seghieri G, Mancini M, Filippelli M, Duranti R, Fabbri A, Innocenti F, Iandelli I, Misuri G. Dyspnoea, peripheral airway involvement and respiratory muscle effort in patients with type I diabetes mellitus under good metabolic control. Clin Sci (Lond) 1999. 96(5):499-506. [DOD] [CrossRef]
- Wanke T, Formanek D, Auinger M, Popp W, Zwick H, Irsigler K. Inspiratory muscle performance and pulmonary function changes in insulin-dependent diabetes mellitus. Am Rev Respir Dis 1991. 143(1):97-100. [DOD]
- Fuso L, Pitocco D, Longobardi A, Zaccardi F, Contu C, Pozzuto C, Basso S, Varone F, Ghirlanda G, Antonelli Incalzi R. Respiratory muscle strength and endurance in type 2 diabetes mellitus. Diabetes Met Res Rev 2012. In press. [DOD]
- Schuyler MR, Niewoehner DE, Inkley SR, Kohn R. Abnormal lung elasticity in juvenile diabetes mellitus. Am Rev Respir Dis 1976. 113(1):37-41. [DOD]
- Ozsahin K, Tugrul A, Mert S, Yüksel M, Tugrul G. Evaluation of pulmonary alveolo-capillary permeability in Type 2 diabetes mellitus: using technetium 99mTc-DTPA aerosol scintigraphy and carbon monoxide diffusion capacity. J Diabetes Complications 2006. 20(4):205-209. [DOD] [CrossRef]
- Dalquen P. The lung in diabetes mellitus. Respiration 1999. 66(1):12-13. [DOD] [CrossRef]
- Forgiarini LA Jr, Kretzmann NA, Porawski M, Dias AS, Marroni NA. Experimental diabetes mellitus: oxidative stress and changes in lung structure. J Bras Pneumol 2009. 35(8):788-791. [DOD]
- Marvisi M, Bartolini L, del Borrello P, Brianti M, Marani G, Guariglia A, Cuomo A. Pulmonary function in non-insulin-dependent diabetes mellitus. Respiration 2001. 68(3):268-272. [DOD] [CrossRef]
- Wheatley CM, Baldi JC, Cassuto NA, Foxx-Lupo WT, Snyder EM. Glycemic control influences lung membrane diffusion and oxygen saturation in exercise-trained subjects with type 1 diabetes: alveolar-capillary membrane conductance in type 1 diabetes. Eur J Appl Physiol 2011. 111(3):567-578. [DOD] [CrossRef]
- Nunn JF (ed). Nunn's applied respiratory physiology. 4th ed., vol. 1, 1993, Oxford: Butterworth-Heinemann Medical, p. 656. [DOD]
- Daly WJ, Giammona ST, Ross JC, Feigenbaum H. Effects of pulmonary vascular congestion on postural changes in the perfusion and filling of the pulmonary vascular bed. J Clin Invest 1964. 43:68-76. [DOD] [CrossRef]
- Roughton FJ, Forster RE. Relative importance of diffusion and chemical reaction rates in determining rate of exchange of gases in the human lung, with special reference to true diffusing capacity of pulmonary membrane and volume of blood in the lung capillaries. J Appl Physiol 1957. 11(2):290-302. [DOD]
- Fuso L, Cotroneo P, Basso S, De Rosa M, Manto A, Ghirlanda G, Pistelli R. Postural variations of pulmonary diffusing capacity in insulin-dependent diabetes mellitus. Chest 1996. 110(4):1009-1013. [DOD] [CrossRef]
- Pitocco D, Santangeli P, Fuso L, Zaccardi F, Longobardi A, Infusino F, Incalzi RA, Lanza GA, Crea F, Ghirlanda G. Association between reduced pulmonary diffusing capacity and cardiac autonomic dysfunction in type 1 diabetes. Diabet Med 2008. 25(11):1366-1369. [DOD]
- Davis WA, Knuiman M, Kendall P, Grange V, Davis TM. Glycemic exposure is associated with reduced pulmonary function in type 2 diabetes: the Fremantle Diabetes Study. Diabetes Care 2004. 27(3):752-757. [DOD] [CrossRef]
- McKeever TM, Weston PJ, Hubbard R, Fogarty A. Lung function and glucose metabolism: an analysis of data from the Third National Health and Nutrition Examination Survey. Am J Epidemiol 2005. 161(6):546-556. [DOD] [CrossRef]
- Lawlor DA, Ebrahim S, Smith GD. Associations of measures of lung function with insulin resistance and Type 2 diabetes: findings from the British Women's Heart and Health Study. Diabetologia 2004. 47(2):195-203. [DOD] [CrossRef]
- Yeh HC, Punjabi NM, Wang NY, Pankow JS, Duncan BB, Cox CE, Selvin E, Brancati FL. Cross-sectional and prospective study of lung function in adults with type 2 diabetes: the Atherosclerosis Risk in Communities (ARIC) study. Diabetes Care 2008. 31(4):741-746. [DOD] [CrossRef]
- Mancini M, Filippelli M, Seghieri G, Iandelli I, Innocenti F, Duranti R, Scano G. Respiratory muscle function and hypoxic ventilatory control in patients with type I diabetes. Chest 1999. 115(6):1553-1562. [DOD] [CrossRef]
- Scano G, Filippelli M, Romagnoli I, Mancini M, Misuri G, Duranti R, Rosi E. Hypoxic and hypercapnic breathlessness in patients with type I diabetes mellitus. Chest 2000. 117(4):960-967. [DOD] [CrossRef]
- van den Borst B, Gosker HR, Zeegers MP, Schols AM. Pulmonary function in diabetes: a metaanalysis. Chest 2010. 138(2):393-406. [DOD] [CrossRef]
- Gribbin J, Hubbard R, Smith C. Role of diabetes mellitus and gastro-oesophageal reflux in the aetiology of idiopathic pulmonary fibrosis. Respir Med 2009. 103(6):927-931. [DOD] [CrossRef]
- Ford ES. Body mass index, diabetes, and C-reactive protein among U.S. adults. Diabetes Care 1999. 22(12):1971-1977. [DOD] [CrossRef]
- Lazarus R, Sparrow D, Weiss ST. Baseline ventilatory function predicts the development of higher levels of fasting insulin and fasting insulin resistance index: the Normative Aging Study. Eur Respir J 1998. 12(3):641-645. [DOD] [CrossRef]
- Engstrom G, Lind P, Hedblad B, Wollmer P, Stavenow L, Janzon L, Lindgarde F. Lung function and cardiovascular risk: relationship with inflammation-sensitive plasma proteins. Circulation 2002. 106(20):2555-2560. [DOD] [CrossRef]
- Haffner SM. Insulin resistance, inflammation, and the prediabetic state. Am J Cardiol 2003. 92(4A):18J-26J. [DOD] [CrossRef]
- Barrett-Connor E, Frette C. NIDDM, impaired glucose tolerance, and pulmonary function in older adults. The Rancho Bernardo Study. Diabetes Care 1996. 19(12):1441-1444. [DOD] [CrossRef]
- Lange P, Groth S, Kastrup J, Mortensen J, Appleyard M, Nyboe J, Jensen G, Schnohr P. Diabetes mellitus, plasma glucose and lung function in a cross-sectional population study. Eur Respir J 1989. 2(1):14-19. [DOD]
- Guazzi M, Oreglia I, Guazzi MD. Insulin improves alveolar-capillary membrane gas conductance in type 2 diabetes. Diabetes Care 2002. 25(10):1802-1806. [DOD] [CrossRef]
- Giordano A, Calcagni ML, Rossi B, Fuso L, Accardo D, Valente S, Pistelli R, Franceschini R, Troncone L. Potential use of iodine-123 metaiodobenzylguanidine radioaerosol as a marker of pulmonary neuroadrenergic function. Eur J Nucl Med 1997. 24(1):52-58. [DOD] [CrossRef]
- Santos e Fonseca CM, Manco JC, Gallo Junior L, Barreira AA, Foss MC. Cholinergic bronchomotor tone and airway caliber in insulin-dependent diabetes mellitus. Chest 1992. 101(4):1038-1043. [DOD] [CrossRef]
- West JB (ed). Respiratory physiology: the essential. 4th ed., 1990, Williams and Wilkins, Baltimore. [DOD]
- Fuso L, Paladini L, Pitocco D, Musella T, Contu C, Maugeri L, Santamaria AP, Varone F, Ghirlanda G, Antonelli Incalzi R. Pulmonary diffusing capacity for carbon monoxide: a marker of depressed hypercapnic drive in type 1 diabetes mellitus? Diabet Med 2011. 28(11):1407-1411. [DOD]
- Komatsu WR, Gabbay MA, Castro ML, Saraiva GL, Chacra AR, de Barros Neto TL, Dib SA. Aerobic exercise capacity in normal adolescents and those with type 1 diabetes mellitus. Pediatr Diabetes 2005. 6(3):145-149. [DOD] [CrossRef]
- Sokolov E, Demidov Iu I, Dudaev VA. Physical work ability in patients with type 1 diabetes. Klin Med (Mosk) 2008. 86(1):54-57. [DOD]
- Baldi JC, Cassuto NA, Foxx-Lupo WT, Wheatley CM, Snyder EM. Glycemic status affects cardiopulmonary exercise response in athletes with type I diabetes. Med Sci Sports Exerc 2010. 42(8):1454-1459. [DOD] [CrossRef]
- Komatsu WR, Barros Neto TL, Chacra AR, Dib SA. Aerobic exercise capacity and pulmonary function in athletes with and without type 1 diabetes. Diabetes Care 2010. 33(12):2555-2557. [DOD] [CrossRef]
- Regensteiner JG, Sippel J, McFarling ET, Wolfel EE, Hiatt WR. Effects of non-insulin-dependent diabetes on oxygen consumption during treadmill exercise. Med Sci Sports Exerc 1995. 27(6):875-881. [DOD]
- Regensteiner JG, et al. Abnormal oxygen uptake kinetic responses in women with type II diabetes mellitus. J Appl Physiol 1998. 85(1):310-317. [DOD]
- Estacio RO, Regensteiner JG, Wolfel EE, Jeffers B, Dickenson M, Schrier RW. The association between diabetic complications and exercise capacity in NIDDM patients. Diabetes Care 1998. 21(2):291-295. [DOD] [CrossRef]
- Vanninen E, Uusitupa M, Siitonen O, Laitinen J, Lansimies E. Habitual physical activity, aerobic capacity and metabolic control in patients with newly-diagnosed type 2 (non-insulin-dependent) diabetes mellitus: effect of 1-year diet and exercise intervention. Diabetologia 1992. 35(4):340-346. [DOD] [CrossRef]
- Dwyer GB, Wallace JP, Whaley MH. Influence of metabolic control on the ventilatory threshold in adults with non insulin-dependent diabetes mellitus. Diabetes Res 1994. 25(1):39-46. [DOD]
- Vanninen E, Uusitupa M, Remes J, Siitonen O, Laitinen J, Länsimies E, Pyörälä K. Relationship between hyperglycaemia and aerobic power in men with newly diagnosed type 2 (non insulin-dependent) diabetes. Clin Physiol 1992. 12(6):667-677. [DOD] [CrossRef]
- Fang ZY, Sharman J, Prins JB, Marwick TH. Determinants of exercise capacity in patients with type 2 diabetes. Diabetes Care 2005. 28(7):1643-1648. [DOD] [CrossRef]
- Brassard P, Ferland A, Bogaty P, Desmeules M, Jobin J, Poirier P. Influence of glycemic control on pulmonary function and heart rate in response to exercise in subjects with type 2 diabetes mellitus. Metabolism 2006. 55(11):1532-1537. [DOD] [CrossRef]
- Lange P, Groth S, Mortensen J, Appleyard M, Nyboe J, Schnohr P, Jensen G. Diabetes mellitus and ventilatory capacity: a five year follow-up study. Eur Respir J 1990. 3(3):288-292. [DOD]
- Lange P, Parner J, Schnohr P, Jensen G. Copenhagen City Heart Study: longitudinal analysis of ventilatory capacity in diabetic and nondiabetic adults. Eur Respir J 2002. 20(6):1406-1412. [DOD] [CrossRef]
- Kerstjens HA, Rijcken B, Schouten JP, Postma DS. Decline of FEV1 by age and smoking status: facts, figures, and fallacies. Thorax 1997. 52(9):820-827. [DOD] [CrossRef]
- Kaminsky DA. Spirometry and diabetes: implications of reduced lung function. Diabetes Care 2004. 27(3):837-838. [DOD] [CrossRef]
- Antonelli Incalzi R, Fuso L, Pitocco D, Basso S, Trove A, Longobardi A, Calcagni ML, Giordano A, Ghirlanda G. Decline of neuroadrenergic bronchial innervation and respiratory function in type 1 diabetes mellitus: a longitudinal study. Diabetes Metab Res Rev 2007. 23(4):311-316. [DOD] [CrossRef]
- Weiss SR, Cheng SL, Kourides IA, Gelfand RA, Landschulz WH. Inhaled insulin provides improved glycemic control in patients with type 2 diabetes mellitus inadequately controlled with oral agents: a randomized controlled trial. Arch Intern Med 2003. 163(19):2277-2282. [DOD] [CrossRef]
- Skyler JS, Weinstock RS, Raskin P, Yale JF, Barrett E, Gerich JE, Gerstein HC. Use of inhaled insulin in a basal/bolus insulin regimen in type 1 diabetic subjects: a 6-month, randomized, comparative trial. Diabetes Care 2005. 28(7):1630-1635. [DOD] [CrossRef]
- Cefalu WT. Concept, strategies, and feasibility of noninvasive insulin delivery. Diabetes Care 2004. 27(1):239-246. [DOD] [CrossRef]
- Hsia CC, Raskin P. The diabetic lung: relevance of alveolar microangiopathy for the use of inhaled insulin. Am J Med 2005. 118(3):205-211. [DOD] [CrossRef]
- Cefalu WT, Skyler JS, Kourides IA, Landschulz WH, Balagtas CC, Cheng S, Gelfand RA. Inhaled human insulin treatment in patients with type 2 diabetes mellitus. Ann Intern Med 2001. 134(3):203-207. [DOD]
- Rosenstock J, Zinman B, Murphy LJ, Clement SC, Moore P, Bowering CK, Hendler R, Lan SP, Cefalu WT. Inhaled insulin improves glycemic control when substituted for or added to oral combination therapy in type 2 diabetes: a randomized, controlled trial. Ann Intern Med 2005. 143(8):549-558. [DOD]
- Fuso L, Pitocco D, Incalzi RA. Inhaled insulin and the lung. Curr Med Chem 2007. 14(12):1335-1347. [DOD] [CrossRef]
- Coughlin SR, Mawdsley L, Mugarza JA, Calverley PM, Wilding JP. Obstructive sleep apnoea is independently associated with an increased prevalence of metabolic syndrome. Eur Heart J 2004. 25:735-741. [DOD] [CrossRef]
- Punjabi NM, Beamer BA. Alterations in glucose disposal in sleep-disordered breathing. Am J Respir Crit Care Med 2009. 179:235-240. [DOD] [CrossRef]
- Punjabi NM, Ahmed MM, Polotsky VY, Beamer BA, O'Donnell CP. Sleep-disordered breathing, glucose intolerance, and insulin resistance. Respir Physiol Neurobiol 2003. 136:167-178. [DOD] [CrossRef]
- West SD, Nicoll DJ, Stradling JR. Prevalence of obstructive sleep apnoea in men with type 2 diabetes. Thorax 2006. 61:945-950. [DOD] [CrossRef]
This article has been cited by other articles:
|
Thorax Computed Tomography Findings in Patients with Erectile Dysfunction
Resorlu M, Arslan M, Karatag O, Adam G
J Clin Imag Sci 2017. 7:25
|
|
|
Diabetes and the lung
Wilkens H, Leser D
Pneumol 2017. 14(4):219-227
|
|
|
The impact of lungs from diabetic donors on lung transplant recipients
Ambur V, Taghavi S, Jayarajan S, Kadakia S, Zhao H, Gomez-Abraham J, Toyoda Y
Eur J Cardiothorac Surg 2017. 51(2):285-290
|
|
|
Potential Biochemical Mechanisms of Lung Injury in Diabetes
Zheng H, Wu J, Jin Z, Yan LJ
Aging Dis 2017. 8(1):7-16
|
|
|
Redox imbalance and mitochondrial abnormalities in the diabetic lung
Wu J, Jin Z, Yan LJ
Redox Biol 2016. In press
|
|
|
ALLOXAN - DIABETES CAUSES OXIDATIVE IMBALANCE AND MORPHOLOGICAL, MORPHOMETRIC AND ULTRASTRUCTURAL CHANGES IN RAT LUNGS
Suarez OA, Lucchesi AN, Cataneo AJ, Spadella CT
Eur J Pharm Med Res 2016. 3(12):1-10
|
|
|
Lung function in patients with diabetes mellitus
Fontaine-Delaruelle C, Viart-Ferber C, Luyton C, Couraud S
Rev Pneumol Clin 2016. 72(1):10-16
|
|
|
Pulmonary Function Test Profile in 8-18 Years Old Patients with Type 1 Diabetes Mellitus
Irlisnia I, Supriyatno B, Tridjadja B, Windiastuti E
Sari Pediatri 2015. 17(4):241-248
|
|
|
The lung endothelin system: a potent therapeutic target with bosentan for the amelioration of lung alterations in a rat model of diabetes mellitus
Cayir A, Ugan RA, Albayrak A, Kose D, Akpinar E, Cayir Y, Atmaca HT, Bayraktutan Z, Kara M
J Endocrinol Invest 2015. 38(9):987-998
|
|
|
High-mobility group box 1 enhances the inflammatory process in diabetic lung
Boteanu RM, Uyy E, Suica VI, Antohe F
Arch Biochem Biophys 2015. 583:55-64
|
|
|
STUDY OF PULMONARY FUNCTION TESTS IN TYPE 2 DIABETI C PATIENTS: CORRELATION WITH GLYCEMIC STATUS
Kulkarni GV, Surdi AD
Int J Pharm Bio Sci 2015. 6(4):138-145
|
|
|
Cystic fibrosis related diabetes
O'Shea D, O'Connell J
Curr Diab Rep 2014. 14(8):511
|
|
|
Selective therapeutic effect of cornus officinalis fruits on the damage of different organs in STZ-induced diabetic rats
Han Y, Jung HW, Park YK
Am J Chin Med 2014. 42(5):1169-1182
|
|
|
Type 2 Diabetes Mellitus: The Concerned Complications and Target Organs
Kumar A, Bharti SK, Kumar A
Apollo Med 2014. 11(3):161-166
|
|
|
Effect of oral vanadium supplementation on oxidative stress factors in the lung tissue of diabetic rats
Yilmaz-Ozden T, Kurt-Sirin O, Tunali S, Akev N, Can A, Yanardag R
Trace Elements Electrol 2014. 31(2):48-52
|
|
|
Effects of vitamin E on pathological changes induced by diabetes in rat lungs
Alireza S, Leila N, Siamak S, Mohammad-Hasan KA, Behrouz I
Respir Physiol Neurobiol 2013. 185(3):593-599
|
|
|
Is lung a target of diabetic injury? The novel pro and cons evidence
Popov D
Proc Rom Acad 2013. 15(2):99-104
|
|
|