Review
Rev Diabet Stud,
2013,
10(2-3):133-156 |
DOI 10.1900/RDS.2013.10.133 |
Endothelial Dysfunction in Diabetes: Pathogenesis, Significance, and Treatment
Sandra J. Hamilton1, Gerald F. Watts2
1Combined Universities Centre for Rural Health, University of Western Australia, Geraldton, Australia
2School of Medicine and Pharmacology, Royal Perth Hospital Unit, University of Western Australia, Perth, Australia
Address correspondence to: Gerald F. Watts, e-mail: gerald.watts@uwa.edu.au
Manuscript submitted March 20, 2013; resubmitted April 15, 2013; accepted June 13, 2013.
Keywords: type 2 diabetes, dyslipoproteinemia, lipoprotein, vascular disease
Abstract
Type 2 diabetes (T2D) markedly increases the risk of cardiovascular disease. Endothelial dysfunction (ED), an early indicator of diabetic vascular disease, is common in T2D and independently predicts cardiovascular risk. Although the precise pathogenic mechanisms for ED in T2D remain unclear, at inception they probably involve uncoupling of both endothelial nitric oxide synthase activity and mitochondrial oxidative phosphorylation, as well as the activation of vascular nicotinamide adenine dinucleotide phosphate oxidase. The major contributing factors include dyslipoproteinemia, oxidative stress, and inflammation. Therapeutic interventions are designed to target these pathophysiological factors that underlie ED. Therapeutic interventions, including lifestyle changes, antiglycemic agents and lipid-regulating therapies, aim to correct hyperglycemia and atherogenic dyslipidemia and to improve ED. However, high residual cardiovascular risk is seen in both research and clinical practice settings. Well-designed studies of endothelial function in appropriately selected volunteers afford a good opportunity to test new therapeutic interventions, paving the way for clinical trials and utilization in the care of the diabetic patient. However, based on the results from a recent clinical trial, niacin should not be added to a statin in individuals with low high-density lipoprotein cholesterol and very well controlled low-density lipoprotein cholesterol.
Abbreviations: ACCORD - Action to Control Cardiovascular Risk in Diabetes; ADMA - asymmetric dimethylarginine; AGE - advanced glycation end-product; Ach - acetylcholine AIM-HIGH - Atherothrombosis Intervention in Metabolic Syndrome with Low HDL-cholesterol/High Triglyceride and Impact on Global Health Outcomes; ApoB - apolipoprotein B; BH4 - tetrahydrobiopterin; CAD - coronary artery disease; CETP - cholesterylester transfer protein; cGMP - cyclic guanosine 3',5'-monophosphate; CHD - coronary heart disease; CoQ10 - coenzyme Q10; CRP - C-reactive protein; CVD - cardiovascular disease; DHA - docosahexaemoic acid; DPP4 - dipeptidyl peptidase 4; ecSOD - extracellular superoxide dismutase; ED - endothelial dysfunction; EDHF - endothelial-derived hyperpolarizing factor; EMP - endothelial-derived microparticle; ET-1 - endothelin-1; eNOS - endothelial nitric oxide synthase; EPA - eicosapentaenoic acid; ER niacin - extended release niacin; FABF - forearm blood flow; FIELD - Fenofibrate Intervention and Event Lowering in Diabetes study; FMD - flow-mediated dilatation; glcNAC - N-acetylglucosamine; GLP - glucagon-like peptide; GLUT4 - glucose transporter; HDL - high-density lipoprotein; HPS2-THRIVE - Heart Protection Study 2 and the Treatment of HDL to Reduce the Incidence of Vascular Events; hs-CRP - high-sensitivity C-reactive protein; ICAM-1 - intercellular adhesion molecule 1; IRS-1 - insulin receptor substrate 1; LDL- low-density lipoprotein; L-NMMA - L-nitro-mono-methyl arginine; Lp(a) - lipoprotein (a); LpB - apolipoprotein B; LpC - apolipoprotein C; Lp-PLA2 - lipoprotein-associated phospholipase A2; MDA - malondialdehyde; MRI - magnetic resonance imaging; NADPH - nicotinamide adenine dinucleotide phosphate; NO - nitric oxide; NOS - nitric oxide synthase; O2- - superoxide; PAT - peripheral arterial tonometry; PCSK9 - proprotein convertase subtilisin/kexin type 9; PKC - protein kinase C; PPARα - Peroxisome proliferator-activated receptor alpha; ROS - reactive oxygen species; SGLT2 - type 2 sodium-glucose cotransporter inhibitors; SNP - single nucleiotide polymorphism; T2D - type 2 diabetes; VCAM-I - vascular cellular adhesion molecule 1; vWF - von Willebrand factor
1. Introduction
Type 2 diabetes (T2D) markedly increases the risk of all forms of cardiovascular disease with prospective studies demonstrating a significantly increased incidence of morbidity and mortality [1-3]. Endothelial dysfunction (ED), an early indicator of diabetic vascular disease, is common in T2D and independently predicts cardiovascular risk [4]. The pathogenesis of ED in diabetes is complex. The major contributing pathophysiological factors include dyslipoproteinemia [5], oxidative stress and inflammation [6-8]. Although important, dysglycemia, hypertension and insulin resistance chiefly operate through oxidative stress and pro-inflammatory pathways [6, 9, 10]. Both invasive and non-invasive methods for assessing endothelial function have generated a wealth of knowledge concerning the pathogenesis and therapeutic regulation of ED in T2D [4, 11, 12]. Treatment strategies necessarily target the pathophysiological factors that underlie ED and range from lifestyle interventions to nutritional supplements and specific pharmacological therapies [12]. We review these areas with a focus on dyslipoproteinemia.
2. Endothelial function
2.1 Normal endothelial function
The endothelium maintains vascular homeostasis through multiple and complex physiological functions, including the release of several vasoactive factors that regulate vascular tone, blood fluidity and coagulation, while limiting smooth muscle cell proliferation and inflammation [11, 13, 14]. Arguably, the most critical endothelium-derived molecule is nitric oxide (NO), although maintenance of endothelial function also involves endothelin-1 (ET-1), angiotensin II, protacyclin, and endothelial-derived hyperpolarizing factor (EDHF) [4].
In response to shear stress or activation of muscarinic receptors, a G-protein signal transduction pathway is activated, stimulating endothelial NO synthase (eNOS) [4]. In a tightly coupled process, this forms NO and citrulline from L-arginine, molecular oxygen and reduced nicotinamide adenine dinucleotide phosphate (NADPH) (Figure 1A) [4, 13]. NO released by this process functions via a cyclic guanosine 3', 5'-monophosphate (cGMP) mediated pathway, resulting in vasodilation and inhibition of chemotaxis and platelet aggregation [13, 15].
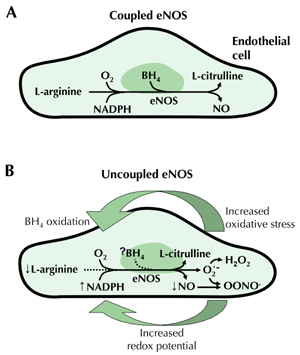 |
 |
Figure 1. A: Nitric oxide (NO) is produced from L-arginine and molecular oxygen (O2) by endothelial nitric oxide synthase (eNOS) in a tightly ‘coupled’ process involving tetrahydrobiopterin (BH4) and nicotinamide adenine dinucleotide phosphate (NADPH). B: In diabetes, increased redox imbalance (due to increased NADH/NADPH) and decreased availability of BH4 (due to oxidation) leads to 'uncoupling' of NO production. This results in transfer of electrons to O2 to form superoxide (O2˙). Superoxide in turn reacts with and consumes NO, forming the oxidant species peroxynitrite (OONO-). Hence, oxidative stress and endothelial dysfunction are further increased [39, 41]. Abbreviations: NADH - reduced nicotinamide adenine dinucleotide, H2O2 - hydrogen peroxide. |
|
2.2 Measurement of endothelial function
Endothelial function may be measured by invasive and non-invasive methods (Table 1). Indirect measures in the peripheral circulation assess the vasodilatory responses of conduit and resistance arteries to stimuli that increase NO release [4, 11, 15, 16]. In the brachial artery, shear stress is generated by hyperemia following an induced period of local ischemia, and flow-mediated dilatation (FMD), an endothelium-dependent function, is measured using high-resolution ultrasonography or even magnetic resonance imaging (MRI) [16-18]. Venous occlusion strain-gauge plethysmography can be utilized to measure blood flow changes in the forearm microcirculation following hyperemia, or intra-arterial infusion of muscarinic receptor agonists such as acetycholine [19, 20]. An emerging non-invasive clinical tool to assess peripheral vasodilator response is digital peripheral arterial tonometry (PAT) (Endo-PAT, Itamar Medical) [21, 22].
Table
1.
Methods of assessing endothelial function |
|
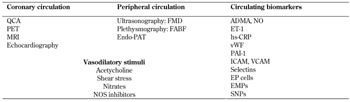 |
 |
Legend:
QCA - quantitative coronary angiography, PET - positron emission tomography, MRI - magnetic resonance imaging, FMD - flow-mediated dilation, FABF - forarm blood flow, Endo-PAT - non-invasive peripheral artery tonometry, NOS - nitric oxide synthase, ADMA - asymmetric dimethylarginine, NO - nitric oxide, ET-1 - endothelin-1, hs-CRP - high-sensitivity C-reactive protein, vWF - von Willebrand factor, PAI-1 - plasminogen activator inhibitor 1, ICAM - intercellular adhesion molecule, VCAM - vascular cell adhesion molecule, EP cells - endothelial progenitor cell, EMP - endothelial-derived microparticle, SNP - single nucleiotide polymorphisms. |
|
Endothelial function in coronary arteries may be assessed, in response to intra-arterial infusion of acetycholine or shear stress stimuli, using quantitative angiography to measure vessel diameter changes [23]. Non-invasive methods such as Doppler echocardiography, positron emission tomography and MRI may be utilized to assess coronary microvascular vasodilatory capacity, but these methods are costly [15].
Circulating biomarkers may be measured as indirect indices of endothelial cell damage, activation and inflammation (Table 1) [24-30]. Impaired mobilization or depletion of endothelial progenitor cells derived from bone marrow are involved in the pathogenesis of ED, and their circulating levels can also be used as a marker of ED [31-34]. A relationship between decreased levels of progenitor cells and increased levels of cell-derived microparticles has been demonstrated in T2D [33]. Endothelial-derived microparticles (EMPs) are small membrane-shed vesicles derived from endothelial cell surfaces under conditions of cellular activation or injury/apoptosis [33, 35]. Thus, circulating EMPs may be potential markers of ED and there may be potential value in utilizing the EMP/progenitor cell ratio [33, 35]. Reduced vascular extracellular superoxide dismutase (ecSOD) activity, the major antioxidant enzyme system of the vessel wall, was closely associated with NO-mediated vasodilation in patients with coronary artery disease, suggesting that reduced ecSOD activity contributes to the reduced bioavailability of NO and ED [36]. However, its utility as a surrogate marker of ED in the clinical setting is limited as its measurement requires the intravenous injection of heparin. Relationships between ED and single nucleotide polymorphisms (SNPs) in the eNOS-3 gene have been demonstrated in conduit arteries but not resistance arteries [37].
3. Endothelial dysfunction
3.1 Pathogenesis of endothelial dysfunction
Endothelial dysfunction reflects an imbalance in the endothelial-derived vasoactive substances that promote vasodilation, importantly NO bioavailability, and vasoconstriction. Decreased bioavailability of NO involves either decreased NO synthesis or increased NO degradation, or both, due to enhanced endothelial production of reactive oxygen species (ROS) [15, 38]. With increased oxidative stress, tetrahydrobiopterin (BH4), a cofactor that tightly regulates NO production, is oxidized resulting in the uncoupling of eNOS and reduced NO production [39-41]. The absence of BH4 perpetuates a cycle of vascular oxidative stress through the transfer of electrons to molecular oxygen, forming oxidant species such as superoxide and peroxynitrite, which further consumes NO and increases oxidative stress (Figure 1B) [15, 39, 40]. Elevated levels of asymmetric dimethylarginine (ADMA), an endogenous inhibitor of eNOS through competition with l-arginine, may further reduce NO production [15].
Endothelial dysfunction may also involve altered levels of vasoconstrictors, such as endothelin-1 and angiotensin II, and other vasodilators such as endothelial-derived hyperpolarizing factor (EDHF) and prostacyclin [15, 38].
3.2 Predictive value of endothelial dysfunction
Several studies in a diverse range of subjects have shown that ED measured by the aforementioned techniques in different vascular beds is predictive of clinical events [42-54]. The principal studies are shown in Table 2. Two recent meta-analyses concluded that FMD is predictive of cardiovascular events and may provide independent prognostic information that is at least equal to the information gained from conventional cardiovascular risk factors; however, future research is required to confirm FMD's efficacy in the assessment of cardiovascular risk [55, 56].
Table
2.
Principal studies evaluating endothelial dysfunction as a predictor of cardiovascular events |
|
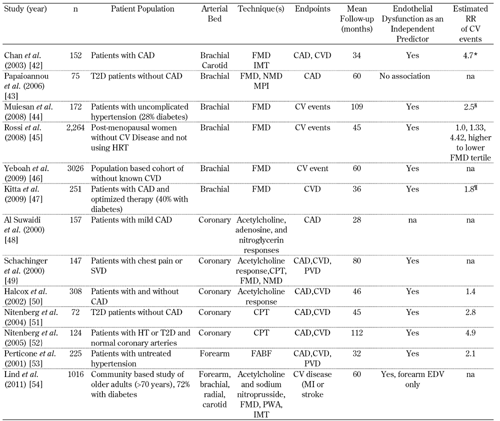 |
 |
Legend:
* Approximation for patients with high IMT plaque burden. § Estimated for patients with impaired FMD compared with those with preserved FMD. ¶ Estimated for patients with persistently impaired FMD compared with those with improved FMD. Abbreviations: RR – relative risk, CV – cardiovascular, T2D – type 2 diabetes mellitus, CAD – coronary artery disease, EDV – endothelial-dependent vasodilation, FMD – flow-mediated dilation of the brachial artery, NMD – nitroglycerin-mediated dilation, MPI – myocardial perfusion imaging, na – not assessed, HT – hypertension, CPT – cold-pressor test, CVD – cerebrovascular disease, IMT – intima medial thickness, FABF – forearm blood flow, PVD – peripheral vascular disease, PWA – pulse wave-based method, SVD – single vessel disease. References [42-54]. |
|
Studies in type 2 diabetic patients have demonstrated endothelial dysfunction to be predictive of cardiovascular outcomes. Coronary endothelial dysfunction, as assessed by the cold-pressor test, was predictive of long-term cardiovascular events in type 2 diabetic patients with normal coronary arteriography [52]. In type 2 diabetic patients without known coronary artery disease (CAD), normal endothelial function, assessed by myocardial perfusion imaging, had a 93% negative predictive value in excluding CAD [43]. In patients with newly diagnosed CAD and impaired brachial artery FMD (43% with diabetes), persistently impaired FMD following 6 months of optimized lifestyle changes and pharmacotherapy was an independent predictor of future cardiovascular events [47]. Impaired endothelial-dependent vasodilation in forearm resistance arteries, but not the brachial artery, was associated with a 5 year risk of major cardiovascular events in a community based study of 1016 older adults (72% with diabetes) [54].
3.3 Endothelial dysfunction in type 2 diabetes
Endothelial dysfunction has been demonstrated in T2D, in both the peripheral and coronary circulation [57-63]. Elevated levels of circulating biomarkers, indirect indices of endothelial cell damage, activation and inflammation, are found in type 2 diabetic patients. These include the soluble adhesion molecules E-selectin, vascular cellular adhesion molecule (VCAM) 1 and intercellular adhesion molecule (ICAM) 1, indices of vascular inflammation [4, 29, 30, 64]. Similarly, increased levels von Willebrand factor (vWF), a measure of endothelial cell damage and activation are reported [4, 30, 64]. Microalbuminuria, an independent predictor of ED, may indicate widespread vascular dysfunction in diabetes [4, 65].
There is a growing body of evidence elucidating the pathogenetic mechanisms underlying the development of ED in T2D. At inception, they probably involve uncoupling of both eNOS activity (Figure 1B) and mitochondrial oxidative phosphorylation (Figure 2), as well as the activation of vascular NADPH oxidase [12, 39, 66]. These three mechanisms essentially result in the uncoupling of eNOS production, increased generation of superoxide (O2._) radicals and overproduction of peroxynitrite [39]. The main factors that combine to cause these biochemical disturbances are dyslipoproteinemia [5], oxidative stress [5, 66], and inflammation [6, 8, 66]. Other clinical factors also play a role, contributing either individually or synergistically to ED in T2D. These include hypertension [67], visceral obesity [68], insulin resistance [6, 69, 70], postprandial hyperlipidemia [71-73], fasting and postprandial hyperglycemia [74-76], and elevated levels of ADMA [38, 77].
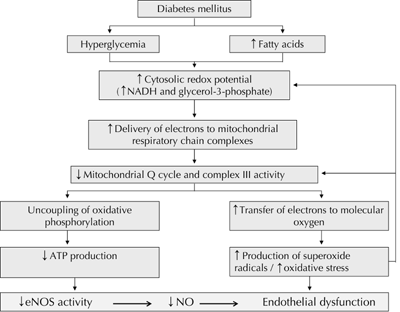 |
 |
Figure 2. Mechanism whereby hyperglycemia and elevated fatty acids induce uncoupling of mitochondrial oxidative phosphorylation and increased oxidative stress in diabetes. Abbreviations: ATP - adenosine triphosphate, NADH - reduced nicotinamide adenine dinucleotide [12]. |
|
Evidence suggests a reciprocal relationship between insulin resistance and ED [6]. The impact of insulin resistance in T2D operates at an insulin signaling level in the vascular endothelium, adipose tissue and skeletal muscle [6]. Impaired insulin receptor substrate-1 (IRS-1) and phosphatidylinositol (PI) 3-kinase in the insulin signaling pathway cause a decreased production of both eNOS and NO resulting in ED on the one hand, and a decreased glucose transporter (GLUT4) translocation in skeletal muscle and adipose tissue leading to peripheral insulin resistance on the other hand [6]. Elevated free fatty acid levels are observed in insulin resistant states, including T2D, obesity and dyslipoproteinemia [5]. This increased fatty acid availability uncouples mitochondrial function in endothelial cells, generating ROS by increasing advanced glycation end-products (AGES), protein kinase C (PKC) and N-acetylglucosamine (glcNAC), impairing eNOS activity and inducing ED [6]. Inflammation, lipotoxicity and glucotoxicity are all increased in diabetes and collectively contribute to insulin resistance and ED [6, 66]. Figure 3 suggests that the pathogenesis of ED in T2D has oxidative stress as the central pathway for a wide spectrum of risk factors [4].
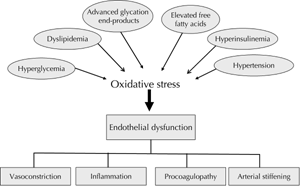 |
 |
Figure 3. Pathogenesis and consequences of endothelial dysfunction in type 2 diabetes mellitus. Oxidative stress contributes to endothelial dysfunction by activating protein kinase C, polyol, hexosamine, and NF kappa B pathways, as well as increasing asymmetric dimethylarginine and advanced glycation end-products [4]. |
|
4. Therapeutic regulation of endothelial dysfunction in type 2 diabetes
Strategies for treating ED in T2D will necessarily target the pathophysiological factors that underlie vasculopathy, such as dyslipidemia, increased oxidative stress, inflammation, visceral obesity, insulin resistance, hyperglycemia, and hypertension [8, 78]. Treatment options range from lifestyle interventions to nutritional supplements and specific pharmacological therapies. We review treatment options, focusing on dyslipoproteinemia.
4.1 Lifestyle interventions
Lifestyle programs aimed at achieving weight loss improve many of the metabolic abnormalities in T2D that contribute to ED, such as hyperglycemia, insulin resistance, visceral obesity, hypertension and dyslipidemia. Weight loss in subjects with the metabolic syndrome improves lipo-protein dysregulation that is associated with obesity [79-82]. Following 4 years of follow-up in the Look AHEAD (Action for Health in Diabetes) study, intensive lifestyle intervention was associated with greater weight loss and improvements in physical fitness [83]. Improvements in glycemic control, blood pressure, triglycerides and HDL-cholesterol were also demonstrated [83]. In type 2 diabetic patients, a reduction in insulin resistance and fat mass following prolonged aerobic exercise resulted in improvements in lipoprotein metabolism [84].
Dietary restriction, weight loss, and increased physical activity have been shown to improve conduit and resistance artery ED in overweight and obese non-diabetic subjects with and without CAD [85-88]. In obese, but otherwise healthy subjects, brachial artery FMD improved with a 6-week low-fat diet but was blunted with a low-carbohydrate diet despite similar degrees of weight loss, suggesting that greater vascular benefit is conferred by a low-fat diet [85]. In obese non-diabetic subjects, improvement in brachial artery FMD following a 6-week low-fat weight loss diet, but not a high-fat weight loss diet, was associated with decreased visceral fat mass and an improved adipokine profile, specifically increased adiponectin and decreased leptin and resistin [89]. Improvement in resistance artery endothelial function following weight loss was mediated by increased NO bioavailability and associated with reductions in abdominal visceral fat [86]. In patients with CAD, greater weight reduction following exercise training was associated with a greater increase in FMD, suggesting a dose response effect [87]. Physical activity also improved plasma markers of endothelial function and oxidative stress in adolescents with the metabolic syndrome [90].
In type 2 diabetic patients, weight loss and increased physical activity have been shown to improve endothelial function [91-93]. An uncontrolled study in obese subjects with insulin resistance syndrome (33% with T2D) demonstrated an improvement in brachial artery FMD and reductions in markers of endothelial activation and coagulation following 6 months of caloric restriction and regular supervised exercise and a 7% mean reduction in body weight [91]. Insulin sensitivity and glycemic control improved and significant increases in HDL-cholesterol levels were demonstrated. In multiple regression analysis, percentage improvement in FMD was strongly associated with percentage weight reduction [91]. A randomized, crossover study of combined aerobic and resistance exercise training for eight weeks demonstrated increased brachial artery FMD and acetylcholine (ACh)-stimulated forearm blood flow (FABF) in T2D subjects [92]. Although glycemic control also improved, reductions in HbA1c and fasting glucose were not correlated with changes in endothelial function [92]. A recent study compared the effects of aerobic exercise, resistance training or usual activities (control group) on endothelial function in women with T2D [93]. Brachial artery FMD was significantly increased by aerobic exercise but not with resistance training or usual activities. Within group weight loss was significantly improved in both the aerobic exercise and resistance training groups but was not correlated with per cent change in FMD. Compared with resistance training and usual activities, aerobic exercise significantly improved HbA1c, but it was not correlated to per cent change in FMD [93]. In a pooled data analysis, the benefits of short-term exercise in subjects with vascular dysfunction were not necessarily dependent on improvement in associated cardiovascular risk factors [94], suggesting that repeated exercise may also act directly on the vasculature via a shear stress-related mechanism, possibly involving endothelial nitric oxide synthase (eNOS) upregulation or reduced NO degradation by free radicals [95].
The association between cigarette smoking and cardiovascular events is supported by a large body of epidemiological evidence [96]. Cigarette smoking is also associated with the premature development of vascular complications in patients with T2D [97]. Cigarette smoke increases inflammation, thrombosis and oxidation of low-density lipoprotein (LDL)-cholesterol, with experimental and clinical evidence supporting the notion that increased oxidative stress results in vascular dysfunction [96]. Both active and passive cigarette smoking are associated with a dose-related impairment of endothelial function [98-100]. Evidence also suggests that endothelial function may improve with smoking cessation [98]. A large prospective randomized, placebo-controlled study investigated the effects of five smoking cessation pharmacotherapies on brachial artery FMD in 1504 current smokers [101]. Smoking intensity was demonstrated to be independently associated with ED [101]. Despite weight gain, endothelial function significantly improved in subjects who quit and remained abstinent at one year, but remained impaired in those who continued to smoke [101]. Studies assessing the effects of smoking cessation in patients with T2D are warranted.
4.2 Lipid-regulating therapy
Lipid regulating therapies target to a greater or lesser degree various aspects of dyslipoproteinemia. Evidence suggests that these therapies correct ED through both lipid lowering and direct effects on the vasculature. The results of selected intervention studies utilizing lipid regulating therapy are reviewed below and summarized in Tables 3 and 4.
Table
3.
Randomized controlled trials investigating the therapeutic regulation of endothelial function in patients with type 2 diabetes mellitus: lipid-regulating monotherapies |
|
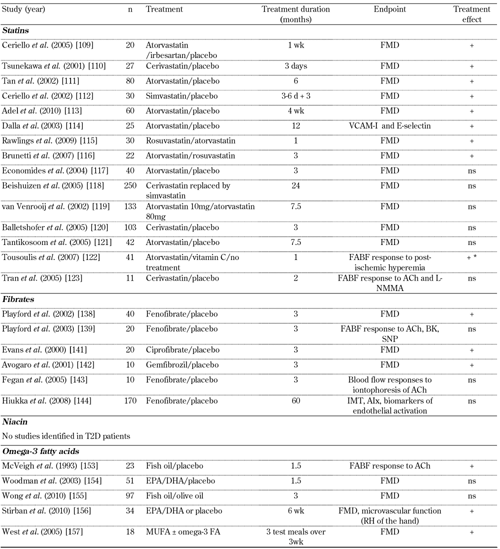 |
 |
Legend:
+ indicates improved endothelial function. ++ indicates combination therapy improved FMD more than monotherapy alone. ± = with or without. * + in atorvastatin only. Abbreviations: FMD – flow-mediated dilation of the brachial artery, ACh – acetylchollilne, FABF – forearm blood flow, ns – no significant effect, SNP – sodium nitroprusside, BK – bradykinin, L-NMMA – L-nitro-mono-methyl arginine, EPA – eicosapentenoic acid, DHA – docosahexenoic acid, T2D – type 2 diabetes mellitus, AIx – augmentation index, IMT – intima-media thickness, MUFA – monounsaturated fatty acids, omega-3 FA – omega-3 fatty acids, CoQ10 – coenzyme Q10, VCAM-I – vascular cell adhesion molecules 1, RH – reactive hyperemia. References [109-123, 138-144, 153-157]. |
|
Table
4.
Randomized controlled trials investigating the therapeutic regulation of endothelial function in patients with type 2 diabetes mellitus: lipid-regulating combination therapies |
|
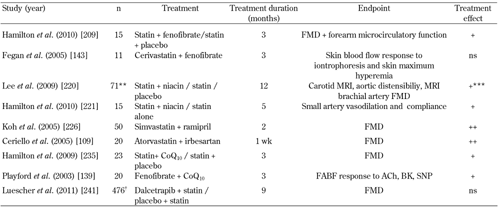 |
 |
Legend:
** only 65% of the patients T2D. *** + in Carotid MRI only. † only 45% of patients T2D. + indicates improved endothelial function. ++ indicates combination therapy improved FMD more than monotherapy alone. Abbreviations: FMD – flow-mediated dilation of the brachial artery, ACh – acetylchollilne, FABF – forearm blood flow, ns – no significant effect, SNP – sodium nitroprusside, BK – bradykinin, L-NMMA – L-nitro-mono-methyl arginine, EPA – eicosapentenoic acid, DHA – docosahexenoic acid, T2D – type 2 diabetes mellitus, AIx – augmentation index, IMT – intima-media thickness, MRI – magnetic resonance imaging, MUFA – monounsaturated fatty acids, omega-3 FA – omega-3 fatty acids, CoQ10 – coenzyme Q10, VCAM-I – vascular cell adhesion molecules 1, RH – reactive hyperemia. References [109, 139, 143, 209, 220, 221, 226, 235, 241]. |
|
Hydroxymethylglutaryl (HMG)-CoA reductase inhibitors (statins). Large clinical studies in a wide range of population subgroups, including subjects with diabetes, have shown statins to reduce cardiovascular mortality [102]. Apart from their main effect in lowering LDL-cholesterol, statins may also have direct anti-inflammatory and antioxidant effects on the vasculature [78]. Although statins improve endothelial function in non-diabetic subjects with dyslipidemia [103, 104], evidence in T2D subjects is inconsistent and contradictory. In uncontrolled studies in T2D subjects, neither serotonin-stimulated FABF nor brachial artery FMD improved with statin therapy [105-108]. In one trial, improvement in endothelial function was demonstrated in a subgroup that attained optimal LDL-lowering [108], but another showed no benefit despite intensive lipid-lowering [106].
A number of randomized, placebo-controlled studies have shown beneficial effects of statins on ED in T2D subjects [109-112]. Improvement in endothelial function occurred within days of treatment, prior to any plasma lipid changes and was correlated with a reduction in oxidative stress, inflammation and endothelial cell activation [109-112]. In normocholesterolemic T2D patients without evidence of CAD, 4 weeks of low dose atrovastatin significantly improved brachial artery FMD compared with placebo [113]. In a placebo-controlled study in T2D patients, atorvastatin was associated with a reduction in vascular cell adhesion molecules (VCAM-I) and E-selectin, suggesting an improvement in endothelial function that was independent of the lipid-regulating effects of atorvastatin [114]. Inhibition of Rho/Rho kinase pathway activity by rosuvastatin or atorvastatin was associated with the improvement in brachial artery FMD in the absence of a reduction in plasma LDL-cholesterol level [115]. In hypertriglyceridemic T2D patients, atorvastatin or rosuvastatin significantly improved FMD and plasma levels of C-reactive protein (CRP), but associations were not reported [116].
However, a number of randomized, double-blind, placebo-controlled studies have shown no effect of statin therapy on ED in subjects with T2D [117, 118], despite improvements in dyslipidemia [119-121]. Forearm vascular reactivity in T2D subjects was improved with atorvastatin [122], but not with cerivastatin [123]. However, ACh-stimulated FABF increased with co-infusion of L-nitro-mono-methyl arginine (L-NMMA), an inhibitor of NOS, suggesting an effect of cerivastatin on non-NO vasoactive mediators, such as EDHF [123].
Emerging LDL-cholesterol lowering therapies. Emerging LDL-cholesterol lowering therapies merit investigation of their vascular effects in T2D patients. Inhibition of proprotein convertase subtilisin/kexin type 9 (PCSK9), an important regulator of the LDL receptor, exerts beneficial effects on LDL and very low-density lipoprotein (VLDL) metabolism, but its role in humans is still to be elucidated [124]. Colesevelam, a more tolerable and potent bile acid sequestrant, is effective at lowering LDL-cholesterol either as monotherapy or in combination with a statin in patients with and without T2D [125, 126]. It has also been demonstrated to improve glycaemic control in T2D patients receiving concurrent antiglycemic therapy [125, 127, 128]. Colesevelam could potentially improve endothelial function by reducing lipotoxicity and glucotoxicity, but no studies reporting its effects on endothelial function are available. Mipomersen, a second-generation antisense oligonucleotide inhibitor of apolipoprotein B (apoB) synthesis, reduces serum apoB, total cholesterol, LDL-cholesterol and lipoprotein(a) (Lp(a)) in a dose dependent manner [129-133]. Studies have demonstrated mipomersen to be an effective lipid-lowering therapy in patients with familial hypercholesterolemia on maximally tolerated statin therapy and in healthy volunteers with mild to moderate hypercholesterolemia [130-133]. Studies in T2D patients are required.
Fibric acid derivatives. A meta-analysis has concluded that fibrates are effective in reducing CVD, primarily by prevention of coronary events [134]. Another recent meta-analysis also suggested that fibrates may be particularly useful in improving dyslipidemia and preventing CVD in people with mild to moderate chronic kidney disease, including diabetics [135]. In addition to their lipid-regulating effects, fibrates may also reduce vascular inflammation and endothelial cell activation. In randomized controlled studies, fibrates appear to have a fairly consistent beneficial effect on endothelial function in subjects with and without T2D. Fenofibrate has been shown to improve brachial artery FMD in statin-naïve non-diabetic subjects with combined hyperlipidemia [136] or primary hypertriglyceridemia [137], and in T2D patients with dyslipidemia [138]. However, fenofibrate alone did not significantly improve forearm microcirculatory function in T2D patients [139]. Moreover, fenofibrate and coenzyme Q10 (CoQ10) independently and interactively lowered 24-h ambulatory blood pressure consistent with their beneficial effects on endothelial function in resistance arterioles [140]. Ciprofibrate and gemfibrozil have been shown to improve brachial artery FMD in type 2 diabetic subjects in fasting and postprandial states [141, 142]. However, fenofibrate therapy in T2D patients did not improve microvascular function when assessed by skin blood flow response to the iontophoresis of acetylcholine [143]. The effects of fenofibrate on markers of oxidative stress and insulin sensitivity are also inconsistent [138, 139, 141].
Although short-term fenofibrate therapy may improve endothelial function [136-138], a sub-study of the longer-term FIELD (Fenofibrate Intervention and Event Lowering in Diabetes) study was not associated with beneficial changes in carotid intima-media thickness, augmentation index, or biomarkers of endothelial function in T2D patients [144]. However, the FIELD study subjects were mostly low risk (as evidenced by the low CVD event rate), and were not selected for having ED at baseline.
Fenofibrate also has beneficial microvascular effects. In FIELD, monotherapy with fenofibrate, significantly reduced the need for laser therapy for diabetic retinopathy compared with placebo [145], and may delay albuminuria progression and impairment of renal function [146]. Recent reports from the Action to Control Cardiovascular Risk in Diabetes (ACCORD) lipid study show that both the addition of fenofibrate to simvastatin and intensive glycemic therapy reduced progression of diabetic retinopathy [147, 148]. In T2D patients with hypertriglyceridemia, adding a fibrate to statin therapy and weight reduction may be safe and effective treatment options for macrovascular and microvascular risk reduction compared with intensification of hypoglycemic and/or statin therapy.
Nicotinic acid (niacin). Niacin may improve endothelial function through direct effects on the vasculature [149]. Compared with placebo, 12 weeks of no-flush niacin (1.5g/day) significantly improved brachial artery FMD in healthy men with low high-density lipoprotein (HDL)-cholesterol (<1.04mmol/l) [150]. However, no change was demonstrated in plasma lipids or chylomicron remnants suggesting a direct vascular effect by niacin [150]. In a placebo-controlled study in metabolic syndrome patients with low HDL-cholesterol (<1.00mmol/l), 52 weeks of extended release niacin (1000mg/day) significantly improved FMD, regressed CIMT, and decreased high sensitivity C-reactive protein (hsCRP). Plasma LDL-cholesterol, HDL-cholesterol and triglycerides were also significantly improved [151]. No studies have reported on patients with T2D. Collectively, these studies demonstrate that niacin is effective in improving endothelial function in subjects with low HDL-cholesterol. Further, improvements in both lipids and inflammatory markers suggest that both lipid-mediated and direct mechanisms are involved in the beneficial vascular effects of niacin.
Omega-3 fatty acids. Omega-3 fatty acids derived from marine fish oil predominantly lower triglycerides, but may also have beneficial effects on HDL subfractions and LDL-particle size, as well as direct actions on the vasculature to reduce inflammation and endothelial cell activation [152]. In randomized, double-blind, controlled trials in T2D patients, omega-3 fatty acid supplementation improved acetylchollilne (Ach)-stimulated FABF [153], but not fasting brachial artery FMD [154, 155]. Compared with placebo, 6 weeks of purified eicosapentenoic acid (EPA)/docosahexemoic acid (DHA) (2g/day) significantly reduced the postprandial decrease in FMD and improved postprandial microvascular function in T2D patients [156]. However, there was no effect on fasting vascular function [156]. In hypertriglyceridemic T2D subjects, inclusion of omega-3 fatty acids in a meal containing predominantly unsaturated fatty acids reduced postprandial lipemia and improved postprandial brachial artery FMD [157], possibly by attenuating the postprandial rise in lipoprotein subclass containing apolipoproteins B and C (LpB:C) [158]. Differential effects of the DHA and EPA components of fish oils were demonstrated in subjects with metabolic syndrome: DHA, but not EPA, enhanced vasodilator responses in the forearm [159].
Probucol. Experimental evidence suggests that probucol may limit oxidative LDL modification and reduce atherogenesis [160]. Probucol reduced coronary restenosis rates following percutaneous transluminal coronary angioplasty [161] and CIMT in patients with hypercholesterolemia [162]. In T2D patients, administration of probucol or atorvastatin decreased urinary 8-hydroxy-2'-deoxyguanosine, a biomarker of overall systemic oxidative stress in vivo, probucol having a greater effect in patients with higher oxidative stress at baseline [163]. Whether probucol improves vascular function and decreases cardiovascular events in patients with T2D requires examination.
In summary, the lipid regulating agents discussed above all correct diabetic dyslipidemia, improving lipid and lipoprotein composition and concentrations to varying degrees and by different mechanisms. Collectively, these agents have been demonstrated to improve ED, but not all the findings are consistent. Endothelial dysfunction is a complex condition that may require a multifactorial strategy to achieve the best cardiovascular outcome.
4.3 Antiglycemic agents and insulin sensitizers
Hyperglycemia contributes to ED by multiple mechanisms, many of which result in increased oxidative stress. Insulin therapy has been shown to improve fasting and postprandial brachial artery FMD [164, 165] and FABF [166-168], possibly through reduced glycaemia and a direct increase in endothelial NO production through 1-phosphatidylinositol 3-kinase signaling [166, 169]. Studies investigating the effects of sulphonylureas on ED have demonstrated inconsistent results in T2D patients [170-172]. A double-blind, randomized, crossover trial suggested that gliclazide, but not glibenclamide, reduced FABF responses to reactive hyperemia, possibly due to differential binding of these agents to sulphonylurea receptors [173]. However, another study did not demonstrate any difference between these two agents on ACh-stimulated FABF in T2D patients [174]. Rapaglinide, a short acting insulin secretagogue, has been shown to improved brachial artery FMD in T2D patients [175] and to ameliorate ED in a glucose dependent manner in subjects with impaired glucose tolerance [176].
Inconsistent vascular effects with metformin treatment have been demonstrated. In a placebo-controlled trial, metformin compared with placebo treatment increased ACh-stimulated FABF and insulin sensitivity in diet-treated T2D patients [177]. In a randomized cross-over study, metformin, but not glimepiride, improved carotid artery diameter and total and systolic blood flow in uncontrolled T2D patients [178]. However, metformin did not improve insulin sensitivity nor ACh-stimulated FABF, despite improved glycemic control, in a randomized, double-blind, placebo-controlled trial in T2D patients [179].
Thiazolidinediones modulate peroxisome proliferator-activated receptor (PPAR) activity and may have direct anti-inflammatory and anti-atherogenic effects on the vasculature through the presence of PPAR-gamma receptors in the endothelium, vascular smooth muscle cells and macrophages [180]. Both troglitazone and pioglitazone increased brachial artery FMD in T2D patients without macrovascular disease [181-183]. However, troglitazone did not improve FMD in T2D patients with more long-standing disease or macrovascular complications [181]. In double-blind, crossover trials, rosiglitazone was shown to increase ACh-stimulated FABF in T2D patients [179, 184].
Acarbose, an alpha-glucosidase inhibitor that targets postprandial hyperglycemia, has been shown to attenuate postprandial impairment of hyperemic FABF response when administered as a single dose in diet-treated T2D patients [185].
Glucagon-like peptide (GLP)-1 is an incretin that decreases glycaemia by stimulating insulin secretion, suppressing glucagon secretion and slowing gastrointestinal motility. GLP-1 may also have a direct modulatory effect on several cardiovascular pathways involved in atherogenesis [186]. Gliptins inhibit dipeptidyl peptidase-4 (DPP4), thereby increasing incretin levels [187]. Infusion of recombinant GLP-1 increased brachial artery FMD in T2D subjects, without any change in insulin resistance [188]. Following a single subcutaneous injection of exenatide (a DPP4 inhibitor) in T2D patients, improved postprandial endothelial function was associated with decreased triglyceride but not glucose concentrations [187]. In a randomized cross-over trial, vildagliptin compared with acarbose improved ACh-stimulated FABF in T2D patients [189].
Pramlintide, a synthetic amylin agonist, lowers postprandial glucose and glucagons, and delays gastric emptying [190]. It is associated with modest improvements in HbA1c levels and weight loss in insulin-requiring T2D patients [190, 191]. Pramlintide also improves cardiovascular risk factors in T2D patients. Modest reductions in triglyceride levels have been reported, and a dose response relationship is suggested [190, 192]. Improvements in markers of inflammation and oxidation have been reported, but there was no significant effect on blood pressure [190, 192].
Future longer-term cardiovascular outcome studies and postprandial arterial function studies, investigating the effects of incretins, gliptins, alpha-glucosidase inhibitors and amylin agonists in statin-treated T2D patients are warranted to establish if their effects translate to improved cardiovascular outcomes.
4.4 Other emerging therapies for T2D
Type 2 sodium-glucose cotransporter inhibitors (SGLT2) have been shown to normalize glycaemia by promoting renal glucose excretion in animal models [193]. Clinical trials in patients with T2D have shown SGLT2 inhibitors to be efficacious in lowering HbA1c, promoting weight loss, having a low incidence of hypoglycemia and complementing other antiglycemic agents [194]. However, correct patient selection and close monitoring after commencement of treatment is advised due to side effects, such as repeated urinary tract infections, genital infections, increased hematocrit and decreased blood pressure [194]. Evidence suggests that succinobucol, a probucol analogue, has protective effects in diabetes via antiatherosclerotic, anti-inflammatory, antioxidant and potential antidiabetic activities [195].
Endothelin 1 (ET-1), a potent vasoconstrictor chronically elevated in T2D, stimulates the production of reactive oxygen species. An experimental model in non-obese moderately diabetic rats showed that the ET-1 system contributes to increased oxidative stress [196]. ETA receptor blockade, but not ETB receptor blockade, abrogated this effect in a dose dependent manner [196]. Coronary microvascular endothelial function improved following 6 months treatment with Atrasentan, a potent and highly selective ETA receptor antagonist, in patients with coronary microvascular endothelial dysfunction on coronary angiography [197].
Experimental inhibition of PKC has been shown to ameliorate functional endothelial resistance and smooth muscle cell hypersensitivity in diabetic hypertensive rats [198]. However, ACh-stimulated endothelial-dependent vasodilation was not restored [198]. Inconsistent effects of PKC beta inhibition have been demonstrated in patients with T2D. Compared with placebo, 6 weeks of treatment with ruboxistaurin, a PKC beta-specific inhibitor, significantly improved brachial artery FMD in patients with T2D [199]. In a placebo-controlled, cross-over design study, 14 days of ruboxistaurin did not change methacholine stimulated (endothelium-dependent) FABF in either T2D patients or healthy control subjects [200].
Future longer-term cardiovascular outcome studies and arterial function studies investigating the effects of these emerging therapies in statin-treated T2D patients are warranted.
4.5 Combination therapies
In large prospective clinical outcome trials, the residual risk of CVD events remains high in T2D patients, despite achievement of optimal or near optimal LDL-cholesterol levels with statin therapy [102, 201-203]. The evidence from studies examining the effects of statins on ED in T2D has been inconsistent and contradictory (Table 3) [109-123]. It is possible that in T2D, treatment with a single therapeutic agent may not adequately improve ED and that combination therapy is required. Several complementary treatment options are possible and reviewed below.
Statins and fibrates. Combination statin and fibrate therapy can significantly benefit dyslipidemia and cardiovascular risk status in T2D patients with combined hyperlipidemia [204-207]. However, evidence for the effects of combined statin/fibrate therapy on ED in T2D patients is limited. In a randomized, double-blind, crossover study, fenofibrate significantly improved endothelial function in the brachial artery and forearm resistance arterioles in statin-treated T2D patients with LDL-Cholesterol <2.6mmol/l and ED [208]. Improvement in brachial artery FMD was inversely associated with on-treatment LDL-cholesterol and apoB concentrations, suggesting that the improvement in endothelial function may in part relate to enhanced reduction in LDL-cholesterol and apoB concentrations [208]. In contrast, microvascular endothelial function was not improved in T2D subjects treated with combination cerivastatin and fenofibrate therapy [143]. Both statin and fibrate therapies have been shown to improve biomarkers of inflammation in subjects with T2D. In 300 subjects with diabetic dyslipidemia, simvastatin or fenofibrate alone or in combination, lowered levels of plasma high sensitivity CR) (hsCRP) and lipoprotein-associated phospholipase A2 (Lp-PLA2). However, there was no additive effect from the combination therapy [209].
Statins and niacins. Nicotinic acid effectively raises HDL-cholesterol, lowers triglycerides and increases LDL particle size [210]. In patients with hyperlipidemia or dyslipidemia, combination niacin extended release/simvastatin therapy resulted in greater favorable changes in size and number of HDL particle subclasses and distribution of HDL particle size compared with atorvastatin alone [211]. In diabetic subjects combination niacin and atorvastatin therapy improves the atherogenic lipid profile more effectively than monotherapy [212].
Combined statin and niacin therapy has been shown to reduce the progression of coronary and carotid atherosclerosis [213-216]. In patients with CAD, the addition of niacin significantly improved endothelial function in patients with low HDL-cholesterol levels [217, 218]. In statin-treated patients with low HDL-cholesterol and CVD (65% T2D with CAD), 12 months of niacin treatment, when compared with placebo, significantly reduced carotid atherosclerosis, but did not alter either aortic distensibility or brachial artery FMD [219]. Statin-treated T2D with LDL-cholesterol <2.5mmol/L and ED were randomized to niacin (nicotinic acid prolonged release) or no additional therapy [220]. Niacin significantly improved small artery vasodilatory function and compliance and reduced serum triglycerides by 47%. An inverse association between maximal forearm post-ischemic blood flow and change in serum triglycerides, suggests that a reduction in triglycerides may in part explain the improvement in endothelial function [220].
However, caution is indicated with the addition of niacin to statins in individuals with low HDL-cholesterol given the recent negative reports from the AIM-HIGH (Atherothrombosis Intervention in Metabolic Syndrome with Low HDL-cholesterol/High Triglyceride and Impact on Global Health Outcomes) study and HPS2-THRIVE (Heart Protection Study-2 and the Treatment of HDL to Reduce the Incidence of Vascular Events). AIM-HIGH was discontinued after 36 months of follow-up due to lack of clinical benefit from the addition of extended release (ER) Niacin to statin (± ezetimibe) therapy, despite significant improvements in HDL-cholesterol and triglycerides [221]. A further consideration in discontinuing AIM-HIGH was an increased stroke risk, but overall this was less than 1% and previous studies have not demonstrated an increased stroke risk with niacin. HPS2-THRIVE compared Tredaptive (ER niacin 2 g plus laropiprant (an antiflushing agent) 40 mg daily) with placebo in 25,673 patients treated with simvastatin plus ezetimibe if required. Following 3.9 years of follow-up, no significant benefit was demonstrated on the primary endpoint of major cardiovascular events and a four-fold greater rate of myopathy was also reported with Tredaptive across all subgroups [222, 223]. Importantly, the safety data showed an excess of new onset diabetes, diabetic complications, infections, bleeding (gastrointestinal and intracranial) and gastrointestinal symptoms with tredaptive. A caveat is that the volunteers were mainly Chinese and had very well controlled LDL-cholesterol (1.6 mmol/l at baseline), so that use of this agent, which has now been withdrawn from the market by Merck, should not be used in people with low HDL cholesterol/high triglyceride and LDL-cholesterol at target. A recent review suggests that the cardiovascular benefits of niacin alone may be independent of effects on HDL-cholesterol [224]. Whether any such benefits from niacin-ER in HPS2-THRIVE were nullified by laropiprant, a prostaglandin D2 inhibitor, remains open to question.
Statins and antihypertensive agents. Statins and antihypertensive agents have differing mechanisms of action on the arterial wall. Therefore, it is possible that in combination they will have an additive and synergistic effect on vascular function [109, 225-227]. Compared to monotherapy, ramipril combined with simvastatin significantly improved FMD and reduced malondialdehyde (MDA) and hs-CRP levels in hypercholesterolemic T2D patients [225]. Adiponectin levels and insulin sensitivity were improved with ramipril alone and combination therapy, but no additive effect was demonstrated with combination therapy [225]. In T2D, postprandial hyperglycemia and hypertriglyceridemia independently and cumulatively decreased FMD and increased biomarkers of inflammation [109]. These detrimental effects were counterbalanced by short-term (1 week) administration of atorvastatin and irbesartan, either alone or in combination; combination therapy being more effective than either monotherapy [109]. Longer-term studies utilizing combined statin and angiotensin receptor blockers in T2D are required. In patients with hypercholesterolemia and hypertension, evidence supports the anti-atherosclerotic effects of combined statin and calcium channel blocker therapy, particularly the combination of amlodipine and atorvastatin [226, 228-230].
Combination therapy with antioxidants. Together with dyslipoproteinemia, increased oxidative stress is a major factor involved in the pathogenesis of ED in T2D. Therefore, supplementation with antioxidants has the potential to improve ED in T2D:
- Statins and antioxidants. In patients with ischemic cardiomyopathy (40% with diabetes), atorvastatin (10mg/day) significantly improved post-ischemic FABF, but this effect was blunted by the co-administration of vitamin E (400 IU/day) [231]. Given the potential for statins to inhibit the cellular synthesis of plasma CoQ10, a by-product of isoprenoid metabolism, their full benefit on improving endothelial function may be blunted [232, 233]. In a randomized, double-blind, crossover study, CoQ10 supplementation significantly improved brachial artery FMD in statin-treated T2D patients with LDL-cholesterol <2.5mmol/l and ED [234]. In patients with CAD (20% with diabetes and 80% statin-treated), CoQ10 supplementation improved ecSOD levels and brachial artery FMD, indicating that the beneficial effects of CoQ10 on endothelial function are in part related to improvements in local vascular oxidative stress [235].
- Fibrates and antioxidants. In dyslipidemic T2D patients with ED, combination fenofibrate and CoQ10 normalized forearm microcirculatory dysfunction [139]. Moreover, fenofibrate and CoQ10 independently and interactively lowered 24-hour ambulatory blood pressure [140], consistent with their beneficial effects on endothelial function in resistance arterioles. This synergistic effect of fenofibrate and CoQ10 may involve co-activation of peroxisome proliferator-activated receptor alpha (PPAR-α) in endothelial and smooth muscle cells, improving the production and action of NO and decreasing the synthesis of endothelin-1.
Other combinations therapies. Other therapies with complementary but differing mechanisms of action have the potential to benefit endothelial function. In T2D patients, aggressive LDL-cholesterol lowering with statins alone or statins plus ezetimibe achieved a similar regression of CIMT in patients who had equivalent LDL-cholesterol reductions [236], but comparative therapeutic effects on endothelial function were not studied. Omega-3 fatty acid supplementation has been shown to improve endothelial function in T2D [153, 156-159], including postprandial endothelial function [156-158]. However, the ORIGIN trial found a lack of cardiovascular benefit with daily supplementation of 1gm of n-3 fatty acids (Omacor) in patients with or at risk for T2D (50% treated with a statin) [237]. The benefit of adding higher dose EPA to statins in hypertriglyceridemic subjects at high CVD risk is currently being tested in the REDUCE-IT trial (A Study of AMR101 to Evaluate Its Ability to Reduce Cardiovascular Events in High Risk Patients with Hypertriglyceridemia and on Statin) [238].
Patients with coronary heart disease (CHD) or CHD risk equivalent, below average HDL-cholesterol and treated with a statin and/or other cholesterol lowering agents to a LDL-cholesterol <2.6mmol/l were randomized in a double-blinded, placebo-controlled study (Dal-VESSEL study) to Dalcetrapib, a cholesterylester transfer protein (CETP) inhibitor, for 36 weeks [239]. Dalcetrapib reduced CETP activity by almost 50% and HDL-cholesterol by 30%, but brachial artery FMD, ambulatory blood pressure and biomarkers of inflammation, oxidative stress and coagulation did not alter with either dalcetrapib or placebo [240]. However, the Dal-PLAQUE study suggested a small trend to improvement in plaque volume [241]; diabetics were not specifically studied and the effect on clinical endpoints must await publication of the Dal-OUTCOMES study. The effect of CETP inhibitors on endothelial function in diabetes warrants investigation.
5. Conclusions
Type 2 diabetic patients are at markedly increased risk of CVD events. Endothelial dysfunction is the earliest manifestation of vascular involvement in diabetes and heralds the increased risk of CVD. Endothelial function may be measured by invasive and non-invasive methods in the coronary and peripheral circulations. In the peripheral circulation ED can be examined indirectly by several non-invasive methods. Studies of ED serve two useful purposes in cardiovascular research. First, they have the potential to identify therapeutic agents that could be tested as monotherapy or combination therapy in clinical endpoint trials. Second, they can help elucidate the mechanisms for the cardiovascular benefits of these treatments. Clinical trials examining the effects of interventions on ED may be limited by subject selection bias, statistical under-powering and technical imprecision in measurements. These factors may account for variation in findings among some of the studies reviewed. As methodologies are refined, measurement of endothelial function could in time provide a practical clinical tool for risk stratifying patients and guiding the intensity of treatments to reverse or prevent progression of cardiovascular disease in diabetes.
Several large clinical trials have demonstrated that statins decrease cardiovascular events in T2D. However, the residual risk of CVD remains high in statin-treated T2D patients. This residual risk may relate to persistent abnormalities in Triglyceride-rich lipoproteins and HDLs which are not fully corrected by statins. Combined therapies have complementary but differing mechanisms of action with the potential to improve atherogenic dyslipidemia and endothelial function. However, not all studies demonstrated a consistently beneficial effect on ED. In our opinion, on the basis of a recent clinical trial, niacin should not be added to a statin in individuals with low high-density lipoprotein cholesterol and very well controlled LDL-cholesterol. Other interventions, such as incretins and amylin agonists, have been shown to reduce triglycerides and improve ED in diabetes, but there is no evidence that they reduce cardiovascular events. These and other new and emerging therapies, such as the LDL-cholesterol lowering agents colesevelam and mipomersen, require investigation in longer term clinical outcome studies. Well-designed studies of endothelial function in appropriately selected volunteers afford a good opportunity to test new therapeutic interventions and their eventual utilization in the care of the diabetic patient.
Disclosures
GFW has received honoraria for Advisory Boards and lectures from the following industry-related companies: Pfizer, Merck Sharpe and Dohme, Solvay, AstraZeneca, GalaxoSmithKilne, Sanofi-Aventis.
References
- Haffner S, Lehto S, Ronnemaa T, Pyorala K, Laakso M. Mortality from coronary heart disease in subjects with type 2 diabetes and in nondiabetic subjects with and without prior myocardial infarction. N Engl J Med 1998. 339(4):229-234. [DOD] [CrossRef]
- Kim J, Koh KK, Quon MJ. The Union of Vascular and Metabolic Actions of Insulin in Sickness and in Health. Arterioscler Thromb Vasc Biol 2005. 25(5):889-891. [DOD] [CrossRef]
- Almdal TD, Scharling HM, Jensen JS, Vestergaard HD. The Independent Effect of Type 2 Diabetes Mellitus on Ischemic Heart Disease, Stroke, and Death: A Population-Based Study of 13,000 Men and Women With 20 Years of Follow-up. Arch Intern Med 2004. 164(13):1422-1426. [DOD] [CrossRef]
- Woodman RJ, Chew GT, Watts GF. Mechanisms, significance and treatment of vascular dysfunction in type 2 diabetes mellitus: focus on lipid-regulating therapy. Drugs 2005. 65(1):31-74. [DOD] [CrossRef]
- Watts GF, Playford DA. Dyslipoproteinaemia and hyperoxidative stress in the pathogenesis of endothelial dysfunction in non-insulin dependent diabetes mellitus: an hypothesis. Atherosclerosis 1998. 141(1):17-30. [DOD] [CrossRef]
- Kim J, Montagnani M, Koh KK, Quon MJ. Reciprocal Relationships Between Insulin Resistance and Endothelial Dysfunction: Molecular and Pathophysiological Mechanisms. Circulation 2006. 113(15):1888-1904. [DOD] [CrossRef]
- Nesto R. C-reactive protein, its role in inflammation, Type 2 diabetes and cardiovascular disease, and the effects of insulin-sensitizing treatment with thiazolidinediones. Diabet Med 2004. 21(8):810-817. [DOD] [CrossRef]
- Dandona P, Aljada A, Chaudhuri A. Endothelial Dysfunction, Inflammation and Diabetes. Rev Endocr Metabol Disord 2004. 5:189-197. [DOD] [CrossRef]
- Versari D, Daghini E, Virdis A. Endothelium-dependent contractions and endothelial dysfunction in human hypertension. Br J Pharmacol 2009. 157:527-536. [DOD] [CrossRef]
- Leiter LA, Lewanczuk RZ. Of the renin-angiotensin system and reactive oxygen species: type 2 diabetes and angiotensin II inhibition. Am J Hypertens 2005. 18(1):121-128. [DOD] [CrossRef]
- Widlansky ME, Gokce N, Keaney JF Jr, Vita JA. The clinical implications of endothelial dysfunction. J Am Coll Cardiol 2003. 42(7):1149-1160. [DOD] [CrossRef]
- Hamilton SJ, Chew GT, Watts GF. Therapeutic regulation of endothelial dysfunction in type 2 diabetes mellitus. Diabetes Vasc Dis Res 2007. 4(2):89-102. [DOD] [CrossRef]
- Esper R, Nordaby R, Vilarino J, Paragano A, Cacharron J, Machado R. Endothelial dysfunction: a comprehensive appraisal. Cardiovas Diabetol 2006. 5(1):4. [DOD] [CrossRef]
- Vita JA, Keaney JF. Endothelial function: a barometer for cardiovascular risk? Circulation 2002. 106(6):640-642. [DOD]
- Levine TB, Levine AB. The endothelium and nitric oxide. Metabolic syndrome and cardiovascular disease 2006. 1st edition, pp 173-210. [DOD]
- Corretti MC, Anderson TJ, Benjamin EJ, Celermajer D, Charbonneau F, Creager MA, Deanfield J, Drexler H, Gerhard-Herman M, Herrington D, et al. Guidelines for the ultrasound assessment of endothelial-dependent flow-mediated vasodilation of the brachial artery: A report of the International Brachial Artery Reactivity Task Force. J Am Coll Cardiol 2002. 39(2):257-265. [DOD] [CrossRef]
- Pyke KE, Tschakovsky ME. The relationship between shear stress and flow-mediated dilatation: implications for the assessment of endothelial function. J Physiol 2005. 568(2):357-369. [DOD] [CrossRef]
- Al-Qaisi M, Kharbanda RK, Mittal TK, Donald AE. Measurement of endothelial funciton and its clinical utility for cardiovascular risk. Vasc Health and Risk Manag 2008. 4(3):647-652. [DOD]
- Higashi Y, Sasaki S, Nakagawa K, Matsuura H, Kajiyama G, Oshima T. A noninvasive measurement of reactive hyperemia that can be used to assess resistance artery endothelial function in humans. Am J Cardiol 2001. 87(1):121-125. [DOD] [CrossRef]
- Benjamin N, Calver A, Collier J, Robinson B, Vallance P, Webb D. Measuring Forearm Blood Flow and Interpreting the Responses to Drugs and Mediators. Hypertension 1995. 25(5):918-923. [DOD] [CrossRef]
- Rubinshtein R, Kuvin JT, Soffler M, Lennon R, Lavi S, Nelson R, Pumper G, Lerman L, Lerman A. Assessment of endothelial function by non-invasive peripheral arterial tonometry predicts late cardiovascular adverse events. Euro Heart J 2010. 31(9):1142-1148. [DOD] [CrossRef]
- Fitch KV, Stavrou E, Looby SE, Hemphill L, Jaff MR, Grinspoon SK. Associations of cardiovascular risk factors with two surrogate markers of subclinical atherosclerosis: Endothelial function and carotid intima media thickness. Atherosclerosis. 2011. 217(2):437-440. [DOD]
- Kato MD, Shiode MD, Yamagata MD, Matsuura MD, Kajiyama MD. Coronary Segmental Responses to Acetylcholine and Bradykinin in Patients With Atherosclerotic Risk Factors. Am J Cardiol 1997. 80(6):751-755. [DOD] [CrossRef]
- Stehouwer CD, Stroes ES, Hackeng WH, Mulder PG, Den Ottolander GJ. von Willebrand factor and development of diabetic nephropathy in IDDM. Diabetes 1991. 40(8):971-976. [DOD] [CrossRef]
- Jensen T, Bjerre-Knudsen J, Feldt-Rasmussen B, Deckert T. Features of endothelial dysfunction in early diabetic nephropathy. Lancet 1989. 1(8636):461-463. [DOD] [CrossRef]
- Gearing AJH, Newman W. Circulating adhesion molecules in disease. Immunol Today. 1993. 14(10):506-512. [DOD]
- Albertini JP, Valensi P, Lormeau B, Aurousseau M, Ferriere F, Attali J, Gattegno L. Elevated concentrations of soluble E-selectin and vascular cell adhesion molecule-1 in NIDDM. Effect of intensive insulin treatment. Diabetes Care 1998. 21(6):1008. [DOD] [CrossRef]
- Hwang SJ, Ballantyne CM, Sharrett AR, Smilth L, Davis C, Gotto A, Boerwinkle E. Circulating adhesion molecules VCAM-1, ICAM-1, and e-selectin in carotid atherosclerosis and incident coronary heart disease cases: The Atherosclerosis Risk In Communities (ARIC) Study. Circulation 1997. 96(12):4219-4225. [DOD] [CrossRef]
- Thorand B, Baumert J, Chambless L, Meisinger C, Kolb H, Doring A, Lowel H, Koenig W, MONICA/KORA Study Group. Elevated markers of endothelial dysfunction predict type 2 diabetes mellitus in middle-aged men and women from the general population. Arterioscler Thromb Vasc Biol 2006. 26(2):398-405. [DOD] [CrossRef]
- Lim SC, Caballero AE, Smakowski P, LoGerfo FW, Horton ES, Veves A. Soluble intercellular adhesion molecule, vascular cell adhesion molecule, and impaired microvascular reactivity are early markers of vasculopathy in type 2 diabetic individuals without microalbuminuria. Diabetes Care 1999. 22(11):1865. [DOD] [CrossRef]
- Hill JM, Zalos G, Halcox JP, Schenke WH, Waclawiw MA, Quyyumi AA, Finkel T. Circulating Endothelial Progenitor Cells, Vascular Function, and Cardiovascular Risk. N Engl J Med 2003. 348(7):593-600. [DOD] [CrossRef]
- Giannotti G, Doerries C, Mocharla PS, Mueller MF, Bahlmann FH, Horyath T, Jiang H, Sorrention SA, Steenken N, Manes C, et al. Impaired Endothelial Repair Capacity of Early Endothelial Progenitor Cells in Prehypertension: Relation to Endothelial Dysfunction. Hypertension 2010. 55(6):1389-1397. [DOD] [CrossRef]
- Curtis AM, Zhang L, Medenilla E, Gui M, Wilkinson P, Hu E, Giri J, Doraiswamy V, Gunda S, Burgert M, et al. Relationship of microparticles to progenitor cells as a measure of vascular health in a diabetic population. Cytometry B Clin Cytom 2010. 78B(5):329-337. [DOD] [CrossRef]
- Avogaro A, Albiero M, Menegazzo L, de Kreutzenberg S, Fadini GP. Endothelial Dysfunction in Diabetes. Diabetes Care 2011. 34(Supplement 2):S285-S290. [DOD] [CrossRef]
- Nozaki T, Sugiyama S, Koga H, Sugamura K, Ohba K, Matsuzawa Y, Sumida H, Matsui K, Jinnouchi H, Ogawa H. Significance of a multiple biomarkers strategy including endothelial dysfunction to improve risk stratification for cardiovascular events in patients at high risk for coronary heart disease. J Am Coll Cardiol 2009. 54(7):601-608. [DOD] [CrossRef]
- Landmesser U, Merten R, Spiekermann S, Buttner K, Drexler H, Hornig B. Vascular extracellular superoxide dismutase activity in patients with coronary artery disease: relation to endothelium-dependent vasodilation. Circulation 2000. 101(19):2264-2270. [DOD] [CrossRef]
- Ingelsson E, Syvänen A, Lind L. Endothelium-dependent vasodilation in conduit and resistance vessels in relation to the endothelial nitric oxide synthase gene. J Hum Hypertens 2008. 22(8):569-578. [DOD] [CrossRef]
- Russo G, Leopold JA, Loscalzo J. Vasoactive substances: Nitric oxide and endothelial dysfunction in atherosclerosis. Vascul Pharmacol 2002. 38(5):259-269. [DOD] [CrossRef]
- Chew GT, Watts GF. Coenzyme Q10 and diabetic endotheliopathy: oxidative stress and the 'recoupling hypothesis'. QJM 2004. 97(8):537-548. [DOD] [CrossRef]
- Alp NJ, Channon KM. Regulation of Endothelial Nitric Oxide Synthase by Tetrahydrobiopterin in Vascular Disease. Arterioscler Thromb Vasc Biol 2004. 24(3):413-420. [DOD] [CrossRef]
- Katusic ZS. Vascular endothelial dysfunction: does tetrahydrobiopterin play a role? Am J Physiol - Heart Circ Physiol 2001. 281(3):H981-H986. [DOD]
- Chan SY, Mancini GB, Kuramoto L, Schulzer M, Frohlich J, Ignaszewski A. The prognostic importance of endothelial dysfunction and carotid atheromaburden in patients with coronary artery disease. J Am Coll Cardiol 2003. 42(6):1037-1043. [DOD] [CrossRef]
- Papaioannou GI, Kasapis C, Seip RL, Grey NJ, Katten D, Wackers FJ, Inzucchi SE, Engel S, Taylor A, Young LH, et al. Value of peripheral vascular endothelial function in the detection of relative myocardial ischemia in asymptomatic type 2 diabetic patients who underwent myocardial perfusion imaging. J Nucl Cardiol 2006. 13(3):362-368. [DOD] [CrossRef]
- Muiesan ML, Salvetti M, Paini A, Monteduro C, Galbassini G, Poisa P, Porteri E, Agabiti-Rosei C, Paderno V, Belotti E, et al. Prognostic role of flow-mediated dilatation of the brachial artery in hypertensive patients. J Hypertens 2008. 26(8):1612-1618. [DOD] [CrossRef]
- Rossi R, Nuzzo A, Origliani G, Modena MG. Prognostic role of flow-mediated dilation and cardiac risk factors in post-menopausal women. J Am Coll Cardiol 2008. 51(10):997-1002. [DOD] [CrossRef]
- Yeboah JM, Folsom AR, Burke GL, Johnson C, Polak JF, Post W, Lima JA, Crouse JR, Herrington DM. Predictive value of brachial flow-mediated dilation for incident cardiovascular events in a population-based study: The Multi-Ethnic Study of Atherosclerosis. Circulation 2009. 120(6):502-509. [DOD] [CrossRef]
- Kitta Y, Obata Je, Nakamura T, Hirano M, Kodama Y, Fujioka D, Saito Y, Kawabata K, Sano K, Kobayashi T, et al. Persistent impairment of endothelial vasomotor function has a negative impact on outcome in patients with coronary artery disease. J Am Coll Cardiol 2009. 53(4):323-330. [DOD] [CrossRef]
- Al Suwaidi J, Hamasaki S, Higano ST, Nishimura RA, Holmes DR Jr, Lerman A. Long-term follow-up of patients with mild coronary artery disease and endothelial dysfunction. Circulation 2000. 101(9):948-954. [DOD] [CrossRef]
- Schachinger VM, Britten MB, Zeiher AM. Prognostic impact of coronary vasodilator dysfunction on adverse long-term outcome of coronary heart disease. Circulation 2000. 101(16):1899-1906. [DOD] [CrossRef]
- Halcox JP, Schenke WH, Zalos GR, Mincemoyer R, Prasad A, Waclawiw MA, Nour KR, Quyyumi AA. Prognostic value of coronary vascular endothelial dysfunction. Circulation 2002. 106(6):653-658. [DOD] [CrossRef]
- Nitenberg A, Valensi P, Sachs R, Cosson E, Attali JR, Antony I. Prognostic value of epicardial coronary artery constriction to the cold pressor test in type 2 diabetic patients with angiographically normal coronary arteries and no other major coronary risk factors. Diabetes Care 2004. 27(1):208-215. [DOD] [CrossRef]
- Nitenberg A, Pham I, Antony I, Valensi P, Attali JR, Chemla D. Cardiovascular outcome of patients with abnormal coronary vasomotion and normal coronary arteriography is worse in type 2 diabetes mellitus than in arterial hypertension: A 10 year follow-up study. Atherosclerosis 2005. 183(1):113-120. [DOD] [CrossRef]
- Perticone F, Ceravolo R, Pujia A, Ventura G, Iacopino S, Scozzafava A, Ferraro A, Chello M, Mastroroberto P, Verdecchia P, Schillaci G. Prognostic Significance of Endothelial Dysfunction in Hypertensive Patients. Circulation 2001. 104(2):191-196. [DOD] [CrossRef]
- Lind L, Berglund L, Larsson A, Sundstrom J. Endothelial function in resistance and conduit arteries and 5-year risk of cardiovascular disease. Circulation 2011. 123(14):1545-1551. [DOD] [CrossRef]
- Inaba Y, Chen J, Bergmann S. Prediction of future cardiovascular outcomes by flow-mediated vasodilatation of brachial artery: a meta-analysis. Int J Cardiovasc Imaging 2010. 26(6):631-640. [DOD] [CrossRef]
- Green DJ, Jones H, Thijssen D, Cable NT, Atkinson G. Flow-mediated dilation and cardiovascular event prediction: does nitric oxide matter? Hypertension 2011. 57(3):363-369. [DOD]
- McVeigh GE, Brennan GM, Johnston GD, McDermott BJ, McGrath LT, Henry WR, Andrews JW, Hayes JR. Impaired endothelium-dependent and independent vasodilation in patients with type 2 (non-insulin-dependent) diabetes mellitus. Diabetologia 1992. 35(8):771-776. [DOD]
- Watts GF, O'Brien SF, Silvester W, Millar JA. Impaired endothelium-dependent and independent dilatation of forearm resistance arteries in men with diet-treated non-insulin-dependent diabetes: role of dyslipidaemia. Clin Sci (Lond) 1996. 91(5):567-573. [DOD]
- Williams SB, Cusco JA, Roddy MA, Johnstone MT, Creager MA. Impaired nitric oxide-mediated vasodilation in patients with non-insulin-dependent diabetes mellitus. J Am Coll Cardiol 1996. 27(3):567-574. [DOD] [CrossRef]
- Enderle MD, Benda N, Schmuelling RM, Haering HU, Pfohl M. Preserved endothelial function in IDDM patients, but not in NIDDM patients, compared with healthy subjects. Diabetes Care 1998. 21(2):271-277. [DOD] [CrossRef]
- Henry RMA, Ferreira I, Kostense PJ, Dekker JM, Nijpels G, Heine RJ, Kamp O, Bouter LM, Stehouwer CD. Type 2 diabetes is associated with impaired endothelium-dependent, flow-mediated dilation, but impaired glucose metabolism is not: The Hoorn Study. Atherosclerosis 2004. 174(1):49-56. [DOD] [CrossRef]
- Nitenberg A, Valensi P, Sachs R, Dali M, Aptecar E, Attali JR. Impairment of coronary vascular reserve and ACh-induced coronary vasodilation in diabetic patients with angiographically normal coronary arteries and normal left ventricular systolic function. Diabetes 1993. 42(7):1017-1025. [DOD] [CrossRef]
- Prior JO, Quinones MJ, Hernandez-Pampaloni M, Facta AD, Schindler TH, Sayre JW, Hsueh WA, Schelbert HR. Coronary Circulatory Dysfunction in Insulin Resistance, Impaired Glucose Tolerance, and Type 2 Diabetes Mellitus. Circulation 2005. 111(18):2291-2298. [DOD] [CrossRef]
- Guerci B, Kearney-Schwartz A, Bohme P, Zannad F, Drouin P. Endothelial dysfunction and type 2 diabetes. Part 1: physiology and methods for exploring the endothelial function. Diabetes Metab 2001. 27(4 Pt 1):425-434. [DOD]
- Papaioannou GI, Seip RL, Grey NJ, Katten D, Taylor A, Inzucchi SE, Young LH, Chyun DA, Davey JA, Wackers FJ, et al. Brachial artery reactivity in asymptomatic patients with type 2 diabetes mellitus and microalbuminuria (from the Detection of Ischemia in Asymptomatic Diabetics-Brachial Artery Reactivity study). Am J Cardiol 2004. 94(3):294-299. [DOD] [CrossRef]
- Tabit C, Chung W, Hamburg N, Vita J. Endothelial dysfunction in diabetes mellitus: Molecular mechanisms and clinical implications. Rev Endocr Metab Disord 2010. 11(1):61-74. [DOD] [CrossRef]
- Iiyama K, Nagano M, Yo Y, Nagano N, Kamide K, Higaki J, Mikami H, Ogihara T. Impaired endothelial function with essential hypertension assessed by ultrasonography. Am Heart J 1996. 132(4):779-782. [DOD] [CrossRef]
- Arcaro G, Zamboni M, Rossi L, Turcato E, Covi G, Armellini F, Bosello O, Lechi A. Body fat distribution predicts the degree of endothelial dysfunction in uncomplicated obesity. Int J Obes 1999. 23(9):936. [DOD] [CrossRef]
- Cleland SJ, Petrie JR, Small M, Elliott HL, Connell JM. Insulin Action Is Associated With Endothelial Function in Hypertension and Type 2 Diabetes. Hypertension 2000. 35(1):507-511. [DOD] [CrossRef]
- Petrie JR, Ueda S, Webb DJ, Elliott HL, Connell JM. Endothelial nitric oxide production and insulin sensitivity: a physiological link with implications for pathogenesis of cardiovascular disease. Circulation 1996. 93(7):1331-1333. [DOD] [CrossRef]
- Nappo F, Esposito K, Cioffi M, Giugliano G, Molinari AM, Paolisso G, Marfella R, Giuglano D. Postprandial endothelial activation in healthy subjects and in type 2 diabetic patients: role of fat and carbohydrate meals. J Am Coll Cardiol 2002. 39(7):1145-1150. [DOD] [CrossRef]
- Anderson RA, Evans ML, Ellis GR, Graham J, Morris K, Jackson SK, Lewis MJ, Rees A, Frenneaux MP. The relationships between postprandial lipaemia, endothelial function and oxidative stress in healthy individuals and patients with type 2 diabetes. Atherosclerosis 2001. 154(2):475-483. [DOD] [CrossRef]
- Anderson RA, Jones CJ, Goodfellow J. Is the fatty meal a trigger for acute coronary syndromes? Atherosclerosis 2001. 159(1):9-15. [DOD]
- Kawano H, Motoyama T, Hirashima O, Hirai N, Miyao Y, Sakamoto T, Kugiyama K, Ogawa H, Yasue H. Hyperglycemia rapidly suppresses flow-mediated endothelium- dependent vasodilation of brachial artery. J Am Coll Cardiol 1999. 34(1):146-154. [DOD] [CrossRef]
- Shige H, Ishikawa T, Suzukawa M, Ito T, Nakajima K, Higashi K, Ayaroi M, Tabata S, Ohsuzu F, Nakamura H. Endothelium-dependent flow-mediated vasodilation in the postprandial state in type 2 diabetes mellitus. Am J Cardiol 1999. 84(10):1272-1274. [DOD] [CrossRef]
- Haller H. Postprandial glucose and vascular disease. Diabet Med 1997. 14(Suppl 3):S50-S56. [DOD]
- Lin KY, Ito AM, Asagami TM, Tsao PS, Adimoolam S, Kimoto M, Tsuji H, Reaven G, Cooke J. Impaired Nitric Oxide Synthase Pathway in Diabetes Mellitus: Role of Asymmetric Dimethylarginine and Dimethylarginine Dimethylaminohydrolase. Circulation 2002. 106(8):987-992. [DOD] [CrossRef]
- Davignon J. Beneficial Cardiovascular Pleiotropic Effects of Statins. Circulation 2004. 109(23 Suppl 1):1-39. [DOD]
- Chan DC, Watts GF, Ng TW, Yamashita S, Barrett PH. Effect of weight loss on markers of triglyceride-rich lipoprotein metabolism in the metabolic syndrome. Eur J Clin Invest 2008. 38(10):743-751. [DOD] [CrossRef]
- Ng TW, Watts GF, Barrett PH, Rye KA, Chan DC. Effect of weight loss on LDL and HDL kinetics in the metabolic syndrome: associations with changes in plasma retinol-binding protein-4 and adiponectin levels. Diabetes Care 2007. 30(11):2945-2950. [DOD] [CrossRef]
- Watts GF, Chan DC. Mechanisms for therapeutic correction of dyslipidaemia in insulin resistance and diabetes. Atheroscler Suppl 2010. 11(1):61-64. [DOD] [CrossRef]
- Chan DC, Watts GF. Dyslipidaemia in the metabolic syndrome and type 2 diabetes: pathogenesis, priorities, pharmacotherapies. Expert Opin Pharmacother 2011. 12(1):13-30. [DOD] [CrossRef]
- Wing R, Look Ahead Research Group. Long-term effects of a lifestyle intervention on weight and cardiovascular risk factors in individuals with type 2 diabetes mellitus: four-year results of the Look AHEAD Trial. Arch Intern Med 2010. 170(17):1566-1575. [DOD]
- Alam S, Stolinski M, Pentecost C, Boroujerdi MA, Jones RH, Sonksen PH, Umpleby AM. The effect of a six-month exercise program on very low-density lipoprotein apolipoprotein B secretion in type 2 diabetes. J Clin Endocrinol Metab 2004. 89(2):688-694. [DOD] [CrossRef]
- Phillips SA, Jurva JW, Syed AQ, Kulinski JP, Pleuss J, Hoffmann RG, Gutterman DD. Benefit of low-fat over low-carbohydrate diet on endothelial health in obesity. Hypertension 2008. 51(2):376-382. [DOD] [CrossRef]
- Pierce GL, Beske SD, Lawson BR, Southall KL, Benay FJ, Donato AJ, Seals DR. Weight loss alone improves conduit and resistance artery endothelial function in young and older overweight/obese adults. Hypertension 2008. 52(1):72-79. [DOD] [CrossRef]
- Ades PA, Savage PD, Lischke S, Toth MJ, Harvey-Berino J, Bunn JY, Ludlow M, Schneider DJ. The effect of weight loss and exercise training on flow-mediated dilatation in coronary heart disease: a randomized trial. Chest 2011. 140(6):1420-1427. [DOD] [CrossRef]
- Bigornia SJ, Mott MM, Hess DT, Apovian CM, McDonnell ME, Duess MA, Kluge MA, Fiscale AJ, Vita JA, Gokce N. Long-term successful weight loss improves vascular endothelial function in severely obese individuals. Obesity (Silver Spring) 2010. 18(4):754-759. [DOD] [CrossRef]
- Varady KA, Bhutani S, Klempel MC, Phillips SA. Improvements in vascular health by a low-fat diet, but not a high-fat diet, are mediated by changes in adipocyte biology. Nutr J 2011. 10:8. [DOD] [CrossRef]
- Camarillo-Romero E, Dominguez-Garcia MV, Amaya-Chavez A, Camarillo-Romero M, Talavera-Pina J, Huitron-Bravo G, Majluf-Cruz A. Effects of a physical activity program on markers of endothelial dysfunction, oxidative stress, and metabolic status in adolescents with metabolic syndrome. ISRN Endocrinol 2012. 2012:970629. [DOD] [CrossRef]
- Hamdy O, Ledbury S, Mullooly C, Jarema C, Porter S, Ovalle K, Moussa A, Caselli A, Caballero AE, Economides PA, et al. Lifestyle Modification Improves Endothelial Function in Obese Subjects With the Insulin Resistance Syndrome. Diabetes Care 2003. 26(7):2119-2125. [DOD] [CrossRef]
- Maiorana A, O'Driscoll G, Cheetham C, Dembo L, Stanton K, Goodman C, Taylor R, Green D. The effect of combined aerobic and resistance exercise training on vascular function in type 2 diabetes. J Am Coll Cardiol 2001. 38(3):860-866. [DOD] [CrossRef]
- Kwon HR, Min KW, Ahn HJ, Seok HG, Lee JH, Park GS, Han KA. Effects of aerobic exercise vs. resistance training on endothelial function in women with type 2 diabetes mellitus. Diabetes Metab J 2011. 35(4):364-373. [DOD] [CrossRef]
- Green DJ, Walsh JH, Maiorana A, Best MJ, Taylor RR, O'Driscoll JG. Exercise-induced improvement in endothelial dysfunction is not mediated by changes in CV risk factors: pooled analysis of diverse patient populations. Am J Physiol - Heart Circ Physiol 2003. 285(6):H2679-H2687. [DOD]
- Green DJ, Maiorana A, O'Driscoll G, Taylor R. Effect of exercise training on endothelium-derived nitric oxide function in humans. J Physiol 2004. 561(1):1-25. [DOD] [CrossRef]
- Ambrose JA, Barua RS. The pathophysiology of cigarette smoking and cardiovascular disease: An update. J Am Coll Cardiol 2004. 43(10):1731-1737. [DOD] [CrossRef]
- Haire-Joshu D, Glasgow RE, Tibbs TL. Smoking and Diabetes. Diabetes Care 2004. 27:S74. [DOD] [CrossRef]
- Celermajer DS, Sorensen KE, Georgakopoulos D, Bull C, Thomas O, Robinaon J, Deanfield JE. Cigarette smoking is associated with dose-related and potentially reversible impairment of endothelium-dependent dilation in healthy young adults. Circulation 1993. 88(5):2149-2155. [DOD] [CrossRef]
- Celermajer DS, Adams MR, Clarkson P, Robinson J, McCredie R, Donald A, Deanfield JE. Passive smoking and impaired endothelium-dependent arterial dilatation in healthy young adults. N Engl J Med 1996. 334(3):150-154. [DOD] [CrossRef]
- Poredos P, Orehek M, Tratnik E. Smoking is associated with dose-related increase of intima-media thickness and endothelial dysfunction. Angiology 1999. 50(3):201-208. [DOD] [CrossRef]
- Johnson HM, Gossett LK, Piper ME, Aeschlimann SE, Korcarz CE, Baker TB, Fiore MC, Stein JH. Effects of Smoking and Smoking Cessation on Endothelial Function: 1-Year Outcomes From a Randomized Clinical Trial. J Am Coll Cardiol 2010. 55(18):1988-1995. [DOD] [CrossRef]
- Collins R, Armitage J, Parish S, Sleigh P, Peto R. MRC/BHF Heart Protection Study of cholesterol-lowering with simvastatin in 5963 people with diabetes: a randomised placebo-controlled trial. Lancet 2003. 361:2005-2016. [DOD] [CrossRef]
- Stroes ES, Koomans HA, de Bruin TW, Rabelink TJ. Vascular function in the forearm of hypercholesterolaemic patients off and on lipid-lowering medication. Lancet 1995. 346:467-471. [DOD] [CrossRef]
- O'Driscoll G, Green D, Taylor R. Simvastatin, an HMG-Coenzyme A Reductase Inhibitor, Improves Endothelial Function Within 1 Month. Circulation 1997. 95(5):1126-1131. [DOD] [CrossRef]
- van de Ree MA, Huisman MV, de Man FH, van der Vijver JC, Meinders AE, Blauw GJ. Impaired endothelium-dependent vasodilation in type 2 diabetes mellitus and the lack of effect of simvastatin. Cardiovasc Res 2001. 52(2):299-305. [DOD]
- van Etten RW, de Koning EJ, Honing ML, Stroes ES, Gaillard CA, Rabelink TJ. Intensive lipid lowering by statin therapy does not improve vasoreactivity in patients with type 2 diabetes. Arterioscler Thromb Vasc Biol 2002. 22(5):799-804. [DOD] [CrossRef]
- Sheu WH, Juang BL, Chen YT, Lee WJ. Endothelial dysfunction is not reversed by simvastatin treatment in type 2 diabetic patients with hypercholesterolemia. Diabetes Care 1999. 22(7):1224-1225. [DOD] [CrossRef]
- Sheu WH, Chen YT, Lee WJ. Improvement in endothelial dysfunction with LDL cholesterol level <80 mg/dl in type 2 diabetic patients. Diabetes Care 2001. 24(8):1499-1501. [DOD] [CrossRef]
- Ceriello A, Assaloni R, Da Ros R, Maier A, Piconi L, Quagliaro L, Esposito K, Giugilano D. Effect of atorvastatin and irbesartan, alone and in combination, on postprandial endothelial dysfunction, oxidative stress, and inflammation in type 2 diabetic patients. Circulation 2005. 111(19):2518-2524. [DOD] [CrossRef]
- Tsunekawa T, Hayashi T, Kano H, Sumi D, Matsui-Hirai H, Thakur NK, Egashira K, Iguchi A. Cerivastatin, a hydroxymethylglutaryl coenzyme A reductase inhibitor, improves endothelial function in elderly diabetic patients within 3 days. Circulation 2001. 104(4):376-379. [DOD] [CrossRef]
- Tan KC, Chow WS, Tam SC, Ai VH, Lam CH, Lam KS. Atorvastatin lowers C-reactive protein and improves endothelium-dependent vasodilation in type 2 diabetes mellitus. J Clin Endocrinol Metab 2002. 87(2):563-568. [DOD] [CrossRef]
- Ceriello A, Taboga C, Tonutti L, Quagliaro L, Piconi L, Bais B, Da Ros R, Motz E. Evidence for an independent and cumulative effect of postprandial hypertriglyceridemia and hyperglycemia on endothelial dysfunction and oxidative stress generation: effects of short- and long-term simvastatin treatment. Circulation 2002. 106(10):1211-1218. [DOD] [CrossRef]
- Adel A, Abdel-Salam Z, Nammas W. Low-dose statin therapy improves endothelial function in type 2 diabetic patients with normal serum total cholesterol: a randomized placebo-controlled study. J Clin Hypertens 2010. 12(10):820-825. [DOD] [CrossRef]
- Dalla N, Passaro A, Zamboni PF, Calzoni F, Fellin R, Solini A. Atorvastatin improves metabolic control and endothelial function in type 2 diabetic patients: a placebo-controlled study. J Endocrinol Invest 2003. 26(1):73-78. [DOD]
- Rawlings R, Nohria A, Liu PY, Donnelly J, Creager MA, Ganz P, Selwyn A, Liao JK. Comparison of effects of rosuvastatin (10 mg) versus atorvastatin (40 mg) on rho kinase activity in Caucasian men with a previous atherosclerotic event. Am J Cardiol 2009. 103(4):437-441. [DOD] [CrossRef]
- Brunetti ND, Maulucci G, Casavecchia GP, Distaso C, De Gennaro L, Pellegrino PL, Di Biase M. Improvement in endothelium dysfunction in diabetics treated with statins: a randomized comparison of atorvastatin 20 mg versus rosuvastatin 10 mg. J Interv Cardiol 2007. 20(6):481-487. [DOD] [CrossRef]
- Economides PA, Caselli A, Tiani E, Khaodhiar L, Horton ES, Veves A. The effects of atorvastatin on endothelial function in diabetic patients and subjects at risk for type 2 diabetes. J Clin Endocrinol Metab 2004. 89(2):740-747. [DOD] [CrossRef]
- Beishuizen ED, Tamsma JT, Jukema JW, van de Ree MA, van der Vijver JC, Meinders AE, Huisman MV. The effect of statin therapy on endothelial function in type 2 diabetes without manifest cardiovascular disease. Diabetes Care 2005. 28(7):1668-1674. [DOD] [CrossRef]
- van Venrooij FV, van de Ree MA, Bots ML, Stolk RP, Huisman MV, Banga JD. Aggressive lipid lowering does not improve endothelial function in type 2 diabetes: the Diabetes Atorvastatin Lipid Intervention (DALI) Study: a randomized, double-blind, placebo-controlled trial. Diabetes Care 2002. 25(7):1211-1216. [DOD] [CrossRef]
- Balletshofer BM, Goebbel S, Rittig K, Enderle M, Schmolzer I, Wascher TC, Ferenc Pap A, Westermeier T, Petzinna D, Matthaei S, Haring HU. Intense cholesterol-lowering therapy with a HMG-CoA reductase inhibitor does not improve nitric oxide dependent endothelial function in type 2 diabetes. Exp Clin Endocrinol Diabetes 2005. 6:324-330. [DOD] [CrossRef]
- Tantikosoom W, Thinkhamrop B, Kiatchusakul S, Jarernsiripornkul N, Srinakarin J, Ojongpian S. Randomized trial of atorvastatin in improving endothelial function in diabetics without prior coronary disease and having average cholesterol level. J Med Assoc Thai 2005. 88(3):399-406. [DOD]
- Tousoulis D, Antoniades C, Vasiliadou C, Kourtellaris P, Koniari K, Marinou K, Charakida M, Ntarladimas I, Siasos G, Stefanadis C. Effects of atorvastatin and vitamin C on forearm hyperaemic blood flow, asymmentrical dimethylarginine levels and the inflammatory process in patients with type 2 diabetes mellitus. Heart 2007. 93(2):244-246. [DOD] [CrossRef]
- Tran D, Lowy A, Howes JB, Howes LG. Effects of cerivastatin on forearm vascular responses, blood pressure responsiveness and ambulatory blood pressure in type 2 diabetic men. Diabetes Obes Metab 2005. 7(3):273-281. [DOD] [CrossRef]
- Chan D. Regulation of proprotein convertase subtilisin/kexin type 9: therapeutical perspectives. Atherosclerosis 2011. 217(1):77-79. [DOD] [CrossRef]
- Goldberg R. Improving glycemic and cholesterol control through an integrated approach incorporating colesevelam - a clinical perspective. Diabetes Metab Syndr Obes 2009. 2:11-21. [DOD] [CrossRef]
- Jialal I, Abby SL, Misir S, Nagendran S. Concomitant reduction in low-density lipoprotein cholesterol and glycated hemoglobin with colesevelam hydrochloride in patients with type 2 diabetes: a pooled analysis. Metab Syndr Relat Disord 2009. 7(3):255-258. [DOD] [CrossRef]
- Fonseca VA, Handelsman Y, Staels B. Colesevelam lowers glucose and lipid levels in type 2 diabetes: the clinical evidence. Diabetes Obes Metab 2010. 12(5):384-392. [DOD] [CrossRef]
- Fonseca VA, Rosenstock J, Wang AC, Truitt KE, Jones MR. Colesevelam HCl improves glycemic control and reduces LDL cholesterol in patients with inadequately controlled type 2 diabetes on sulfonylurea-based therapy. Diabetes Care 2008. 31(8):1479-1484. [DOD] [CrossRef]
- Kastelein JJ, Wedel MK, Baker BF, Su J, Bradley J, Yu R, Chuang E, Graham M, Crooke R. Potent reduction of apolipoprotein B and low-density lipoprotein cholesterol by short-term administration of an antisense inhibitor of apolipoprotein B. Circulation 2006. 114(16):1729-1735. [DOD] [CrossRef]
- Akdim F, Visser ME, Tribble DL, Baker BF, Stroes ES, Yu R, Flaim JD, Su J, Stein EA, Kastelein JJ. Effect of mipomersen, an apolipoprotein B synthesis inhibitor, on low-density lipoprotein cholesterol in patients with familial hypercholesterolemia. Am J Cardiol 2010. 105(10):1413-1419. [DOD] [CrossRef]
- Raal FJ, Santos RD, Blom DJ, Marais AD, Charng M, Cromwell WC, Lachmann RH, Gaudet D, Tan JL, Chasan-Taber S, et al. Mipomersen, an apolipoprotein B synthesis inhibitor, for lowering of LDL cholesterol concentrations in patients with homozygous familial hypercholesterolaemia: a randomised, double-blind, placebo-controlled trial. Lancet 2010. 375(9719):998-1006. [DOD]
- Bell DA, Hooper AJ, Burnett JR. Mipomersen, an antisense apolipoprotein B synthesis inhibitor. Expert Opin Investig Drugs 2011. 20(2):265-272. [DOD] [CrossRef]
- Bell DA, Hooper AJ, Watts GF, Burnett JR. Mipomersen and other therapies for the treatment of severe familial hypercholesterolemia. Vasc Health Risk Manag 2012. 8:651-659. [DOD]
- Jun M, Foote C, Lv J, Neal B, Patel A, Nicholls SJ, Grobbee DE, Cass A, Chalmers J, Perkovic V. Effects of fibrates on cardiovascular outcomes: a systematic review and meta-analysis. Lancet 2010. 375(9729):1875-1884. [DOD] [CrossRef]
- Jun M, Zhu B, Tonelli M, Jardine MJ, Patel A, Neal B, Liyanage T, Keech A, Cass A, Perkovic V. Effects of fibrates in kidney disease: a systematic review and meta-analysis. J Am Coll Cardiol 2012. 60(20):2061-2071. [DOD] [CrossRef]
- Malik J, Melenovsky V, Wichterle D, Haas T, Simek J, Ceska R, Hradec J. Both fenofibrate and atorvastatin improve vascular reactivity in combined hyperlipidaemia (fenofibrate versus atorvastatin trial--FAT). Cardiovasc Res 2001. 52(2):290-298. [DOD] [CrossRef]
- Koh KK, Han SH, Quon MJ, Yeal Ahn J, Shin EK. Beneficial Effects of Fenofibrate to Improve Endothelial Dysfunction and Raise Adiponectin Levels in Patients With Primary Hypertriglyceridemia. Diabetes Care 2005. 28(6):1419-1424. [DOD] [CrossRef]
- Playford DA, Watts GF, Best JD, Burke V. Effect of fenofibrate on brachial artery flow-mediated dilatation in type 2 diabetes mellitus. Am J Cardiol 2002. 90(11):1254-1257. [DOD] [CrossRef]
- Playford DA, Watts GF, Croft KD, Burke V. Combined effect of coenzyme Q10 and fenofibrate on forearm microcirculatory function in type 2 diabetes. Atherosclerosis 2003. 168(1):169-179. [DOD] [CrossRef]
- Chew GT, Watts GF, Davis TM, Stuckey BG, Beilin LJ, Thompson PL, Burke B, Currie PJ. Hemodynamic effects of fenofibrate and coenzyme Q10 in type 2 diabetic subjects with left ventricular diastolic dysfunction. Diabetes Care 2008. 31(8):1502-1509. [DOD] [CrossRef]
- Evans M, Anderson RA, Graham J, Ellis GR, Morris K, Davies S, Jackson SK, Lewis MJ, Frenneaux MP, Rees A. Ciprofibrate Therapy Improves Endothelial Function and Reduces Postprandial Lipemia and Oxidative Stress in Type 2 Diabetes Mellitus. Circulation 2000. 101(15):1773-1779. [DOD] [CrossRef]
- Avogaro A, Miola M, Favaro A, Gottardo L, Pacini G, Manzato E, Zambon S, Sacerdoti D, de Kreutzenberg S, Piliego T, et al. Gemfibrozil improves insulin sensitivity and flow-mediated vasodilatation in type 2 diabetic patients. Eur J Clin Invest 2001. 31(7):603-609. [DOD] [CrossRef]
- Fegan PG, Shore AC, Mawson D, Tooke JE, MacLeod KM. Microvascular endothelial function in subjects with Type 2 diabetes and the effect of lipid-lowering therapy. Diabet Med 2005. 22(12):1670-1676. [DOD] [CrossRef]
- Hiukka A, Westerbacka J, Leinonen ES, Watanabe H, Wiklund O, Hulten LM, Salonen JT, Tuomainen TP, Yki-Jarvinen H, Keech AC, Taskinen MR. Long-term effects of fenofibrate on carotid intima-media thickness and augmentation index in subjects with type 2 diabetes mellitus. J Am Coll Cardiol 2008. 52(25):2190-2197. [DOD] [CrossRef]
- Keech AC, Mitchell P, Summanen PA, O'Day J, Davis TM, Moffitt MS, Taskinen MR, Simes RJ, Tse D, Williamson E, et al. Effect of fenofibrate on the need for laser treatment for diabetic retinopathy (FIELD study): a randomised controlled trial. Lancet 2007. 370(9600):1687-1697. [DOD] [CrossRef]
- Davis T, Ting R, Best J, Donoghoe M, Drury P, Sullivan D, Jenkins A, O'Connell R, Whiting M, Glasziou P, et al. Effects of fenofibrate on renal function in patients with type 2 diabetes mellitus: the Fenofibrate Intervention and Event Lowering in Diabetes (FIELD) Study. Diabetologia 2011. 54(2):280-290. [DOD] [CrossRef]
- Ismail-Beigi F, Craven T, Banerji MA, Basile J, Calles J, Cohen RM, Cuddihy R, Cushman WC, Genuth S, Grimm RH, et al. Effect of intensive treatment of hyperglycaemia on microvascular outcomes in type 2 diabetes: an analysis of the ACCORD randomised trial. Lancet 2010. 376(9739):419-430. [DOD] [CrossRef]
- Chew E, Ambrosius W, Davis M, Danis R, Gangaputra S, Greven C, Hubbard L, Esser BA, Lovato JF, Perdue LH, et al. for the ACCORD Study Group and ACCORD Eye Study Group. Effects of Medical Therapies on Retinopathy Progression in Type 2 Diabetes. N Engl J Med 2010. 363(3):233-244. [DOD] [CrossRef]
- Vosper H. Niacin: a re-emerging pharmaceutical for the treatment of dyslipidaemia. Br J Pharmacol 2009. 158:429-441. [DOD]
- Benjo AM, Maranhao RC, Coimbra SR, Andrade AC, Favarato D, Molina MS, Brandizzi LI, da Luz PL. Accumulation of chylomicron remnants and impaired vascular reactivity occur in subjects with isolated low HDL cholesterol: Effects of niacin treatment. Atherosclerosis 2006. 187(1):116-122. [DOD] [CrossRef]
- Thoenes M, Oguchi A, Nagamia S, Vaccari CS, Hammoud R, Umpierrez GE, Khan BV. The effects of extended-release niacin on carotid intimal media thickness, endothelial function and inflammatory markers in patients with the metabolic syndrome. Int J Clin Pract 2007. 61(11):1942-1948. [DOD] [CrossRef]
- Mori TA, Woodman RJ. The independent effects of eicosapentaenoic acid and docosahexaenoic acid on cardiovascular risk factors in humans. Curr Opin Clin Nutr Metab Care. 2006. 9(2):95-104. [DOD]
- McVeigh GE, Brennan GM, Johnston GD, McDermott BJ, McGrath LT, Henry WR, Andrews JW, Hayes JR. Dietary fish oil augments nitric oxide production or release in patients with Type 2 (non-insulin-dependent) diabetes mellitus. Diabetologia 1993. V36(1):33-38. [DOD] [CrossRef]
- Woodman RJ, Mori TA, Burke V, Puddey IB, Barden A, Watts GF, Beilin LJ. Effects of purified eicosapentaenoic acid and docosahexaenoic acid on platelet, fibrinolytic and vascular function in hypertensive type 2 diabetic patients. Atherosclerosis 2003. 166(1):85-93. [DOD] [CrossRef]
- Wong CY, Yiu KH, Li SW, Lee S, Tam S, Lau CP, Tse HF. Fish-oil supplement has neutral effects on vascular and metabolic function but improves renal function in patients with Type 2 diabetes mellitus. Diabet Med 2010. 27(1):54-60. [DOD] [CrossRef]
- Stirban A, Nandrean S, Gotting C, Tamler R, Pop A, Negrean M, Gawlowski T, Stratmann B, Tschoepe D. Effects of n-3 fatty acids on macro- and microvascular function in subjects with type 2 diabetes mellitus. Am J Clin Nutr 2010. 91(3):808-813. [DOD] [CrossRef]
- West SG, Hecker KD, Mustad VA, Nicholson S, Schoemer SL, Wagner P, Hiderliter AL, Ulbrecht J, Ruey P, Kris-Etherton PM. Acute effects of monounsaturated fatty acids with and without omega-3 fatty acids on vascular reactivity in individuals with type 2 diabetes. Diabetologia 2005. 48(1):113-122. [DOD] [CrossRef]
- Hilpert KF, West SG, Kris-Etherton PM, Hecker KD, Simpson NM, Alaupovic P. Postprandial effect of n-3 polyunsaturated fatty acids on apolipoprotein B-containing lipoproteins and vascular reactivity in type 2 diabetes. Am J Clin Nutr 2007. 85(2):369-376. [DOD]
- Mori TA, Watts GF, Burke V, Hilme E, Puddey IB, Beilin LJ. Differential effects of eicosapentaenoic acid and docosahexaenoic acid on vascular reactivity of the forearm microcirculation in hyperlipidemic, overweight men. Circulation 2000. 102(11):1264-1269. [DOD] [CrossRef]
- Parthasarathy S, Young SG, Witztum JL, Pittman RC. Probucol inhibits oxidative modification of low density lipoprotein. J Clin Invest 1986. 77:641-644. [DOD] [CrossRef]
- Yokoi H, Daida H, Kuwabara Y, Nishikawa H, Takatsu F, Tomihara H, Nakata Y, Kutsumi Y, Ohshima S, Nishiyama S, et al. Effectiveness of an antioxidant in preventing restenosis after percutaneous transluminal coronary angioplasty: the Probucol Angioplasty Restenosis Trial. J Am Coll Cardiol 1997. 30(4):855-862. [DOD] [CrossRef]
- Sawayama Y, Shimizu C, Maeda N, Tatsukawa M, Kinukawa N, Koyanagi S, Kashiwagi S, Hayashi J. Effects of probucol and pravastatin on common carotid atherosclerosis in patients with asymptomatic hypercholesterolemia: Fukuoka atherosclerosis trial (FAST). J Am Coll Cardiol 2002. 39(4):610-616. [DOD] [CrossRef]
- Endo K, Miyashita Y, Sasaki H, Ebisumo M, Ohira M, Saiki A, Koide N, Oyama T, Takeyoshi M, Shirai K. Probucol and atorvastatin decrease urinary 8-hydroxy-2'-deoxyguanosine in patients with diabetes and hypercholesterolemia. J Atheroscler Thromb 2006. 13(1):68-75. [DOD] [CrossRef]
- Evans M, Anderson RA, Smith JC, Khan N, Graham JM, thomas AW, Morris K, Deely D, Frenneaux MP, Davies JS, Rees A. Effects of insulin lispro and chronic vitamin C therapy on postprandial lipaemia, oxidative stress and endothelial function in patients with type 2 diabetes mellitus. Eur J Clin Invest 2003. 33(3):231-238. [DOD] [CrossRef]
- Gaenzer H, Neumayr G, Marschang P, Strum W, Lechleitner M, Foger B, Kirchmair R, Patsch J. Effect of insulin therapy on endothelium-dependent dilation in type 2 diabetes mellitus. Am J Cardiol 2002. 89(4):431-434. [DOD] [CrossRef]
- Rask-Madsen C, Ihlemann N, Krarup T, Christiansen E, Kober L, Kistorp CN, Torp-Pedersen C. Insulin therapy improves insulin-stimulated endothelial function in patients with type 2 diabetes and ischemic heart disease. Diabetes 2001. 50(11):2611-2618. [DOD] [CrossRef]
- Vehkavaara S, Yki-Jarvinen H. 3.5 years of insulin therapy with insulin glargine improves in vivo endothelial function in type 2 diabetes. Arterioscler Thromb Vasc Biol 2004. 24(2):325-330. [DOD] [CrossRef]
- Vehkavaara S, Makimattila S, Schlenzka A, Vakkilainen J, Westerbacka J, Yki-Jarvinen H. Insulin therapy improves endothelial function in type 2 diabetes. Arterioscler Thromb Vasc Biol 2000.20(2):545-550. [DOD] [CrossRef]
- Rask-Madsen C, King GL. Mechanisms of disease: endothelial dysfunction in insulin resistance and diabetes. Nat Clin Pract Endocrinol Metab 2007. 3(1):46-56. [DOD] [CrossRef]
- Sundaresan P, Lykos D, Daher A, Diamond T, Morris R, Howes LG. Comparative effects of glibenclamide and metformin on ambulatory blood pressure and cardiovascular reactivity in NIDDM. Diabetes Care 1997. 20(5):692-697. [DOD] [CrossRef]
- Williams S, Abbott D, Morfis L, Manwaring P, Diamond T, Howes LG. Effects of glibenclamide on blood pressure and cardiovascular responsiveness in non-insulin dependent diabetes mellitus. J Hypertens 1998. 16(5):705-711. [DOD] [CrossRef]
- Spallarossa P, Schiavo M, Rossettin P, Cordone S, Olivotti L, Cordera R, Brunelli C. Sulfonylurea treatment of type 2 diabetic patients does not reduce the vasodilator response to ischemia. Diabetes Care 2001. 24(4):738-742. [DOD] [CrossRef]
- Wascher TC, Boes U. Forearm vascular reactivity is differentially influenced by gliclazide and glibenclamide in chronically treated type 2 diabetic patients. Clin Physiol Funct Imaging 2005. 25(1):40-46. [DOD] [CrossRef]
- Abbink EJ, Pickkers P, Van Rosendaal AJ, Lutterman JA, Tack CJ, Russel FG, Smits P. Vascular effects of glibenclamide vs. glimepiride and metformin in Type 2 diabetic patients. Diabet Med 2002. 19(2):136-143. [DOD] [CrossRef]
- Manzella D, Grella R, Abbatecola AM, Paolisso G. Repaglinide administration improves brachial reactivity in type 2 diabetic patients. Diabetes Care 2005. 28(2):366-371. [DOD] [CrossRef]
- Schmoelzer I, Wascjer TC. Effect of repaglinide on endothelial dysfunction during a glucose tolerance test in subjects with impaired glucose tolerance. Cardiovasc Diabetol 2006. 5(9):4. [DOD]
- Mather KJ, Verma S, Anderson TJ. Improved endothelial function with metformin in type 2 diabetes mellitus. J Am Coll Cardiol 2001. 37(5):1344-1350. [DOD] [CrossRef]
- Machado HA, Vieira M, Cunha MR, Correia MR, Fukui RT, dos Santos RF, Rocha DM, Wajchengerg BL, Lage SG, da Silva ME. Metformin, but not glimepiride, improves carotid artery diameter and blood flow in patients with type 2 diabetes mellitus. Clinics (Sao Paulo) 2012. 67(7):711-717. [DOD] [CrossRef]
- Natali A, Baldeweg S, Toschi E, Capaldo B, Barbaro D, Gastaldelli A, Yudkin JS, Ferrannini E. Vascular effects of improving metabolic control with metformin or rosiglitazone in type 2 diabetes. Diabetes Care 2004. 27(6):1349-1357. [DOD] [CrossRef]
- Gervois P, Fruchart JC, Staels B. Drug Insight: mechanisms of action and therapeutic applications for agonists of peroxisome proliferator-activated receptors. Nat Clin Pract Endocrinol Metab 2007. 3(2):145-156. [DOD] [CrossRef]
- Caballero AE, Saouaf R, Lim SC, Hamdy O, Abou-Elenin K, O'Connor C, Logerfo FW, Horton ES, Veves A. The effects of troglitazone, an insulin-sensitizing agent, on the endothelial function in early and late type 2 diabetes: A placebo-controlled randomized clinical trial. Metabolism 2003. 52(2):173-180. [DOD] [CrossRef]
- Suzuki M, Takamisawa I, Yoshimasa Y, Harano Y. Association between insulin resistance and endothelial dysfunction in type 2 diabetes and the effects of pioglitazone. Diabetes Res Clin 2007. 76(1):12-17. [DOD] [CrossRef]
- Martens FM, Visseren FL, de Koning EJ, Rabelink TJ. Short-term pioglitazone treatment improves vascular function irrespective of metabolic changes in patients with type 2 diabetes. J Cardiovasc Pharmacol 2005. 46(6):773-778. [DOD]
- Pistrosch F, Passauer J, Fischer S, Fuecker K, Hanefeld M, Gross P. In type 2 diabetes, rosiglitazone therapy for insulin resistance ameliorates endothelial dysfunction independent of glucose control. Diabetes Care 2004. 27(2):484-490. [DOD] [CrossRef]
- Shimabukuro M, Higa N, Chinen I, Yamakawa K, Takasu N. Effects of a single administration of acarbose on postprandial glucose excursion and endothelial dysfunction in type 2 diabetic patients: a randomized crossover study. J Clin Endocrinol Metab 2006. 91(3):837-842. [DOD] [CrossRef]
- Forst T, Weber MM, Pfutzner A. Cardiovascular benefits of GLP-1-based therapies in patients with diabetes mellitus type 2: effects on endothelial and vascular dysfunction beyond glycemic control. Exp Diabetes Res 2012. 2012:635472. [DOD] [CrossRef]
- Koska JM, Schwartz EP, Mullin MB, Schwenke DP, Reaven PM. Improvement of postprandial endothelial function after a single dose of exenatide in individuals with impaired glucose tolerance and recent-onset type 2 diabetes. Diabetes Care 2010. 33(5):1028-1030. [DOD] [CrossRef]
- Nystrom T, Gutniak MK, Zhang Q, Zhang F, Holst JJ, Ahren B, Sjoholm A. Effects of glucagon-like peptide-1 on endothelial function in type 2 diabetes patients with stable coronary artery disease. Am J Physiol Endocrinol Metab 2004. 287(6):E1209-E1215. [DOD] [CrossRef]
- van Poppel PC, Netea MG, Smits P, Tack CJ. Vildagliptin improves endothelium-dependent vasodilatation in type 2 diabetes. Diabetes Care 2011. 34(9):2072-2077. [DOD] [CrossRef]
- Hoogwerf BJ, Doshi KB, Diab D. Pramlintide, the synthetic analogue of amylin: physiology, pathophysiology, and effects on glycemic control, body weight, and selected biomarkers of vascular risk. Vasc Health and Risk Manag 2008. 4(2):355-362. [DOD]
- Ryan G, Briscoe TA, Jobe L. Review of pramlintide as adjunctive therapy in treatment of type 1 and type 2 diabetes. Drug Des Devel Ther 2008. 2:203-214. [DOD] [CrossRef]
- Wysham C, Lush C, Zhang B, Maier H, Wilhelm K. Effect of pramlintide as an adjunct to basal insulin on markers of cardiovascular risk in patients with type 2 diabetes. Curr Med Res Opin 2007. 24(1):79-85. [DOD] [CrossRef]
- Hardman TC, Dubrey SW. Development and potential role of type-2 sodium-glucose transporter inhibitors for management of type 2 diabetes. Diabetes Ther 2011. 2(3):133-145. [DOD] [CrossRef]
- Kim Y, Babu AR. Clinical potential of sodium-glucose cotransporter 2 inhibitors in the management of type 2 diabetes. Diabetes Metab Syndr Obes 2012. 5:313-327. [DOD]
- Stocker R. Molecular mechanisms underlying the antiatherosclerotic and antidiabetic effects of probucol, succinobucol, and other probucol analogues. Curr Opin Lipidol 2009. 20(3):227-235. [DOD] [CrossRef]
- Elgebaly MM, Portik-Dobos V, Sachidanandam K, Rychly D, Malcom D, Johnson M, Ergul A. Differential effects of ETA and ETB receptor antagonism on oxidative stress in type 2 diabetes. Vascul Pharmacol 2007. 47(2-3):125-130. [DOD] [CrossRef]
- Reriani M, Raichlin E, Prasad A, Mathew V, Pumper GM, Nelson RE, Lennon R, Rihal C, Lerman LO, Lerman A. Long-term administration of endothelin receptor antagonist improves coronary endothelial function in patients with early atherosclerosis. Circulation 2010. 122(10):958-966. [DOD] [CrossRef]
- Lu X, Bean JS, Kassab GS, Rekhter MD. Protein kinase C inhibition ameliorates functional endothelial insulin resistance and vascular smooth muscle cell hypersensitivity to insulin in diabetic hypertensive rats. Cardiovasc Diabetol 2011. 10:48. [DOD] [CrossRef]
- Mehta NN, Sheetz M, Price K, Comiskey L, Amrutia S, Iqbal N, Mohler ER, Reilly MP. Selective PKC beta inhibition with ruboxistaurin and endothelial function in type-2 diabetes mellitus. Cardiovasc Drugs Ther 2009. 23(1):17-24. [DOD] [CrossRef]
- Beckman JA, Goldfine AB, Goldin A, Prsic A, Kim S, Creager MA. Inhibition of protein kinase C beta does not improve endothelial function in type 2 diabetes. J Clin Endocrinol Metab 2010. 95(8):3783-3787. [DOD] [CrossRef]
- Colhoun PH, Betteridge PD, Durrington PP, Hitaman G, Neil HA, Livingstone SJ, Thomason MJ, Mackness MI, Charlton-Menys V, Fuller J. Primary prevention of cardiovascular disease with atorvastatin in type 2 diabetes in the Collaborative Atorvastatin Diabetes Study (CARDS): multicentre randomised placebo-controlled trial. Lancet 2004. 364(9435):685-696. [DOD] [CrossRef]
- Shepherd J, Barter P, Carema R, Deedwania P, Fruchart J, Haffner S, Hsia J, Breazna A, Larosa J, Grundy S, et al. Effect of lowering LDL cholesterol substantially below currently recommended levels in patients with coronary heart disease and diabetes: The Treating to New Targets (TNT) study. Diabetes Care 2006. 29(6):1220-1226. [DOD] [CrossRef]
- Kearney PM, Blackwell L, Collins R, Keech A, Simes J, Peto R, Armitage J, Baigent C. Efficacy of cholesterol-lowering therapy in 18,686 people with diabetes in 14 randomised trials of statins: a meta-analysis. Lancet 2008. 371:117-125. [DOD] [CrossRef]
- Athyros VG, Papageorgiou AA, Athyrou VV, Demitriadis DS, Kontopoulos AG. Atorvastatin and micronized fenofibrate alone and in combination in type 2 diabetes with combined hyperlipidemia. Diabetes Care 2002. 25(7):1198-1202. [DOD] [CrossRef]
- Durrington PN, Tuomilehto J, Hamann A, Kallend D, Smith K. Rosuvastatin and fenofibrate alone and in combination in type 2 diabetes patients with combined hyperlipidaemia. Diabetes Res Clin Pract 2004. 64(2):137-151. [DOD] [CrossRef]
- Gavish D, Leibovitz E, Shapira I, Rubinstein A. Bezafibrate and simvastatin combination therapy for diabetic dyslipidaemia: efficacy and safety. J Intern Med 2000. 247(5):563-569. [DOD] [CrossRef]
- Derosa G, Cicero AE, Bertone G, Piccinni MN, Ciccarelli L, Roggeri DE. Comparison of fluvastatin plus fenofibrate combination therapyand fluvastatin monotherapy in the treatment of combined hyperlipidemia,type 2 diabetes mellitus, and coronary heart disease: a 12-month, randomized, double-blind, controlled trial. Clin Ther 2004. 26(10):1599-1607. [DOD] [CrossRef]
- Hamilton SJ, Chew GT, Davis TM, Watts GF. Fenofibrate improves endothelial function in the brachial artery and forearm resistance arterioles of statin-treated type 2 diabetic patients. Clin Sci (Lond) 2010. 118(10):607-615. [DOD] [CrossRef]
- Muhlestein JB, May HT, Jensen JR, Horne BD, Lanman RB, Lavasani F, Wolfert RL, Pearson RR, Yannicelli HD, Anderson JL. The reduction of inflammatory biomarkers by statin, fibrate, and combination therapy among diabetic patients with mixed dyslipidemia: The DIACOR (Diabetes and Combined Lipid Therapy Regimen) Study. J Am Coll Cardiol 2006. 48(2):396-401. [DOD] [CrossRef]
- Shepherd J, Betteridge J, Van Gaal L. Nicotinic acid in the management of dyslipidaemia associated with diabetes and metabolic syndrome: a position paper developed by a European Consensus Panel. Curr Med Res Opin 2005. 21(5):665-682. [DOD] [CrossRef]
- Toth PP, Thakker KM, Jiang P, Padley RJ. Niacin extended-release/simvastatin combination therapy produces larger favorable changes in high-density lipoprotein particles than atorvastatin monotherapy. Vasc Health Risk Manag 2012. 8:39-44. [DOD] [CrossRef]
- Van JT, Pan J, Wasty T, Chan E, Wu X, Charles MA. Comparison of extended-release niacin and atorvastatin monotherapies and combination treatment of the atherogenic lipid profile in diabetes mellitus. Am J Cardiol 2002. 89(11):1306-1308. [DOD] [CrossRef]
- Brown BG, Zhao X, Chait A, Fisher LD, Cheung MC, Morse JS, Dowdy AA, Marino EK, Bolson EL, Alaupovic P. Simvastatin and niacin, antioxidant vitamins, or the combination for the prevention of coronary disease. N Engl J Med 2001. 345(22):1583-1592. [DOD] [CrossRef]
- Taylor AJ, Sullenberger LE, Lee HJ, Lee JK, Grace KA. Arterial biology for the investigation of the treatment effects of reducing cholesterol (ARBITER) 2: a double-blind, placebo-controlled study of extended-release niacin on atherosclerosis progression in secondary prevention patients treated with statins. Circulation 2004. 110(23):3512-3517. [DOD] [CrossRef]
- Taylor A, Lee H, Sullenberger L. The effect of 24 months of combination statin and extended-release niacin on carotid intima-media thickness: ARBITER 3. Curr Med Res Opin 2006. 22(11):2243-2250. [DOD] [CrossRef]
- Taylor AJ, Villines TC, Stanek EJ, Devine PJ, Griffen L, Miller M, Weissman NJ, Turco M. Extended-release niacin or ezetimibe and carotid intima-media thickness. N Engl J Med 2009. 361(22):2113-2122. [DOD] [CrossRef]
- Kuvin JT, Ramet ME, Patel AR, Pandian NG, Mendelsohn ME, Karas RH. A novel mechanism for the beneficial vascular effects of high-density lipoprotein cholesterol: Enhanced vasorelaxation and increased endothelial nitric oxide synthase expression. Am Heart J 2002. 144(1):165-172. [DOD] [CrossRef]
- Warnholtz A, Wild P, Ostad MA, Elsner V, stieber F, Schinzel R, Walter U, Peetz D, Lackner K, Blankenberg S, Munzel T. Effects of oral niacin on endothelial dysfunction in patients with coronary artery disease: Results of the randomized, double-blind, placebo-controlled INEF study. Atherosclerosis 2009. 204(1):216-221. [DOD] [CrossRef]
- Lee JM, Robson MD, Yu LM, Shirodaria CC, Cunnington C, Kylintireas I, Digby JE, Bannister T, Handa A, Wiesmann F, et al. Effects of high-dose modified-release nicotinic acid on atherosclerosis and vascular function: a randomized, placebo-controlled, magnetic resonance imaging study. J Am Coll Cardiol 2009. 54(19):1787-1794. [DOD] [CrossRef]
- Hamilton SJ, Chew GT, Davis TM, Watts GF. Niacin improves small artery vasodilatory function and compliance in statin-treated type 2 diabetic patients. Diab Vasc Dis Res 2010. 7(4):296-299. [DOD] [CrossRef]
- Boden WE, Probstfield JK, Anderson T, Chaitman BR, Koprowicz K, Teo K, Weintraub W, et al. Niacin in patients with low HDL cholesterol levels receiving intensive statin therapy. N Engl J Med 2011. 365(24):2255-2267. [DOD] [CrossRef]
- HPS2-THRIVE Collaborative Group. HPS2-THRIVE randomized placebo-controlled trial in 25 673 high-risk patients of ER niacin/laropiprant: trial design, pre-specified muscle and liver outcomes, and reasons for stopping study treatment. Eur Heart J 2013. 34:1279-1291. [DOD] [CrossRef]
- HPS-2 THRIVE misses primary end point: no benefit of niacin/laropiprant. http://www.theheart.org/article/1490635.do. [DOD]
- Lavigne PM, Karas RH. The current state of niacin in cardiovascular disease prevention: a systematic review and meta-regression. J Am Coll Cardiol 2013. 61(4):440-446. [DOD] [CrossRef]
- Koh KK, Quon MJ, Han SH, Ahn JY, Jin DK, Kim JS, Kim DS, Shin EK. Vascular and metabolic effects of combined therapy with ramipril and simvastatin in patients with type 2 diabetes. Hypertension 2005. 45(6):1088-1093. [DOD] [CrossRef]
- Martin-Ventura JL, Tunon J, Duran MC, Blanco-Colio LM, Vivanco F, Egido J. Vascular protection of dual therapy (atorvastatin-amlodipine) in hypertensive patients. J Am Soc Nephrol 2006. 17(12 Suppl 3):S189-S193. [DOD] [CrossRef]
- Koh KK, Quon MJ, Han SH, Ahn JY, Lee Y, Shin EK. Combined therapy with ramipril and simvastatin has beneficial additive effects on tissue factor activity and prothrombin fragment 1+2 in patients with type 2 diabetes. Atherosclerosis 2007. 194(1):230-237. [DOD] [CrossRef]
- Jukema JW, Zwinderman AH, van Boven AJ, Reiber JH, Van der Laarse A, Lie KI, Bruschke AV. Evidence for a Synergistic Effect of Calcium Channel Blockers With Lipid-Lowering Therapy in Retarding Progression of Coronary Atherosclerosis in Symptomatic Patients With Normal to Moderately Raised Cholesterol Levels. Arterioscler Thromb Vasc Biol 1996. 16(3):425-430. [DOD] [CrossRef]
- Jukema JW, Ad JB, Aeilko HZ, Arnoud VD, Albert VG. Proposed synergistic effect of calcium channel blockers with lipid-lowering therapy in retarding progression of coronary atherosclerosis. Cardiovasc Drugs and Ther 1998. 12:111-118. [DOD] [CrossRef]
- Fogari R, Derosa G, Lazzari P, Zoppi A, Fogari E, Rinaldi A, Mugellini A. Effect of amlodipine-atorvastatin combination on fibrinolysis in hypertensive hypercholesterolemic patients with insulin resistance. Am J Hypertens 2004. 17(9):823-827. [DOD] [CrossRef]
- Tousoulis D, Antoniades C, Vassiliadou C, Toutouza M, Pitsavos C, Tentolouris C, Trikas A, Stefanadis C. Effects of combined administration of low dose atorvastatin and vitamin E on inflammatory markers and endothelial function in patients with heart failure. Eur J Heart Fail 2005. 7(7):1126-1132. [DOD] [CrossRef]
- Watts GF, Castelluccio C, Rice-Evans C, Taub NA, Baum H, Quinn PJ. Plasma coenzyme Q (ubiquinone) concentrations in patients treated with simvastatin. J Clin Pathol 1993. 46(11):1055-1057. [DOD] [CrossRef]
- Littarru GP, Langsjoen P. Coenzyme Q10 and statins: Biochemical and clinical implications. Mitochondrion 2007. 7(Supplement 1):S168-S174. [DOD] [CrossRef]
- Hamilton SJ, Chew GT, Watts GF. Coenzyme Q10 improves endothelial dysfunction in statin-treated type 2 diabetic patients. Diabetes Care 2009. 32(5):810-812. [DOD] [CrossRef]
- Tiano L, Belardinelli R, Carnevali P, Principi F, Seddaiu G, Littarru GP. Effect of coenzyme Q10 administration on endothelial function and extracellular superoxide dismutase in patients with ischaemic heart disease: a double-blind, randomized controlled study. Eur Heart J 2007. 28(18):2249. [DOD] [CrossRef]
- Fleg JL, Mete M, Howard BV, Umans JG, roman MJ, Ratner RE, Silverman A, Galloway JM, Henderson JA, Weir MR, et al. Effect of statins alone versus statins plus ezetimibe on carotid atherosclerosis in type 2 diabetes: The SANDS (Stop Atherosclerosis in Native Diabetics Study) Trial. J Am Coll Cardiol 2008. 52(25):2198-2205. [DOD] [CrossRef]
- Bosch J, Gerstein HC, Dagenais GR, Diaz R, Dyal L, Jung H, Maggiono AP, Probstfield J, Riddle MC, Ryden LE, et al. n-3 fatty acids and cardiovascular outcomes in patients with dysglycemia. N Engl J Med 2012. 367(4):309-318. [DOD] [CrossRef]
- Clinical Trials.gov. A Study of AMR101 to evaluate its ability to reduce cardiovascular events in high risk patients with hypertriglyceridemia and on statin (REDUCE-IT). http://clinicaltrials.gov/show/NCT01492361. [DOD]
- Kastelein JJP, Duivenvoorden R, Deanfield J, do Groot E, Judema JW, Kaski J, Munzel T, Taddei S, Lehnert V, Burgess T, et al. Rationale and design of dal-VESSEL: a study to assess the safety and efficacy of dalcetrapib on endothelial function using brachial artery flow-mediated vasodilatation. Curr Med Res Opin 2011. 27(1):141-150. [DOD] [CrossRef]
- Luescher TF, Taddei S, Kaski J, Jukema JW, Kallend D, Munzel t, Kastelein JJ, Deanfield JE, dal-VESSEL investigators. dal-VESSEL: Efficacy and safety of dalcetrapib in patients with or at risk of coronary heart disease - the dal-VESSEL trial. Conference abstract. http://www.escardio.org/congresses/esc-2011/congress-reports/Pages/706-3-dal-VESSEL.aspx#presenter. Accessed 13/01/12. [DOD]
- Fayad ZA, Mani V, Woodward M, Kallend D, Abt M, Burgess T, Fuster V, Ballantyne CM, Stein EA, Tardif J, et al. Safety and efficacy of dalcetrapib on atherosclerotic disease using novel non-invasive multimodality imaging (dal-PLAQUE): a randomised clinical trial. Lancet 2011. 378:1547-1559. [DOD] [CrossRef]
This article has been cited by other articles:
|
Activation of thromboxane A2 receptors mediates endothelial dysfunction in diabetic mice
Xie X, Sun W, Wang J, Li X, Liu X, Liu N
Clin Exp Hypertens 2017. 17:1-7
|
|
|
Associations of hyperglycemia and insulin resistance with biomarkers of endothelial dysfunction in Hispanic/Latino youths: Results from the Hispanic Community Children's Health Study/Study of Latino Youth (SOL Youth)
Parrinello CM, Hua S, Carnethon MR, Gallo LC, Hudson BI, Goldberg RB, Delamater AM, Kaplan RC, Isasi CR
J Diabetes Complications 2017. 31(5):836-842
|
|
|
Impaired endothelial function and arterial stiffness in patients with type 2 diabetes - The effect of a very low-dose combination of fluvastatin and valsartan
Boncelj Svetek M, Erzen B, Kanc K, Sabovic M
J Diabetes Complications 2017. 31(3):544-550
|
|
|
Central Role of Metabolism in Endothelial Cell Function and Vascular Disease
Bierhansl L, Conradi LC, Treps L, Dewerchin M, Carmeliet P
Physiology (Bethesda) 2017. 32(2):126-140
|
|
|
Chronic administration of atorvastatin could partially ameliorate erectile function in streptozotocin-induced diabetic rats
Park J, Kwon OS, Cho SY, Paick JS, Kim SW
Plos One 2017. 12(2):e0172751
|
|
|
Proanthocyanidins from Vitis vinifera inhibit oxidative stress-induced vascular impairment in pulmonary arteries from diabetic rats
Pinna C, Morazzoni P, Sala A
Phytomedicine 2017. 25:39-44
|
|
|
Resveratrol rescues hyperglycemia-induced endothelial dysfunction via activation of Akt
Li JY, Huang WQ, Tu RH, Zhong GQ, Luo BB, He Y
Acta Pharmacol Sin 2017. 38(2):182-191
|
|
|
Analysis of Serum Endothelial Cell-Specific Molecule 1 (Endocan) Level in Type 2 Diabetes Mellitus With Acute ST-Segment Elevation Myocardial Infarction and its Correlation: A Pilot Study
Qiu CR, Fu Q, Sui J, Zhang Q, Wei P, Wu Y, Zhu K, Lu Y, Wan P
Angiology 2017. 68(1):74-78
|
|
|
The nuclear factor (erythroid-derived 2)-like 2 (Nrf2) activator dh404 protects against diabetes-induced endothelial dysfunction
Sharma A, Rizky L, Stefanovic N, Tate M, Ritchie RH, Ward KW, de Haan JB
Cardiovasc Diabetol 2017. 16(1):33
|
|
|
Synergistic effects of metformin with liraglutide against endothelial dysfunction through GLP-1 receptor and PKA signalling pathway
Ke J, Liu Y, Yang J, Lu R, Tian Q, Hou W, Wang G, Wei R, Hong T
Sci Rep 2017. 7:41085
|
|
|
Cerebral microbleeds, cognitive impairment, and MRI in patients with diabetes mellitus
Zhou H, Yang J, Xie P, Dong Y, You Y, Liu J
Clin Chim Acta 2017. 470:14-19
|
|
|
Effect of Pleurotus Ostreatus on the endothelial dysfunction in ovariectomized diabetic rats
El-Bana MA, Fathy SA, Moneim MM, Ezz MK, Metwaly HH
Biosci Res 2017. 14(1):104-113
|
|
|
Pattern of adhesive molecules expression in placenta of non-complicated ART pregnancies
Abdel-Hamid AA, Firgany AE, Mesbah Y, Soliman MF
Placenta 2016. 48:126-132
|
|
|
Early drug development: assessment of proarrhythmic risk and cardiovascular safety
Lester RM, Olbertz J
Expert Rev Clin Pharmacol 2016. 9(12):1611-1618
|
|
|
Arginase Inhibition Improves Microvascular Endothelial Function in Patients With Type 2 Diabetes Mellitus
Kövamees O, Shemyakin A, Checa A, Wheelock CE, Lundberg JO, Östenson CG, Pernow J
J Clin Endocrinol Metab 2016. 101(11):3952-3958
|
|
|
The relationship of systemic markers of renal function and vascular function with retinal blood vessel responses
Heitmar R, Varma C, De P, Lau YC, Blann AD
Graefes Arch Clin Exp Ophthalmol 2016. 254(11):2257-2265
|
|
|
Pancreatic perfusion and subsequent response to glucose in healthy individuals and patients with type 1 diabetes
Carlbom L, Espes D, Lubberink M, Eriksson O, Johansson L, Jansson L, Korsgren O, Ahlström H, Carlsson PO
Diabetologia 2016. 59(9):1968-1972
|
|
|
Diabetes and ageing-induced vascular inflammation
Assar ME, Angulo J, Rodriguez-Manas L
J Physiol 2016. 594(8):2125-2146
|
|
|
Resveratrol via activation of LKB1-AMPK signaling suppresses oxidative stress to prevent endothelial dysfunction in diabetic mice
Hu M, Liu B
Clin Exp Hypertens 2016. 38(4):381-387
|
|
|
Down-Regulation of MicroRNA-137 Improves High Glucose-Induced Oxidative Stress Injury in Human Umbilical Vein Endothelial Cells by Up-Regulation of AMPKα1
Li J, Li J, Wei T, Li J
Cell Physiol Biochem 2016. 39(3):847-859
|
|
|
Astaxanthin alleviates oxidative stress insults-related derangements in human vascular endothelial cells exposed to glucose fluctuations
Abdelzaher LA, Imaizumi T, Suzuki T, Tomita K, Takashina M, Hattori Y
Life Sci 2016. 150:24-31
|
|
|
Evidence for elevated (LIMK2 and CFL1) and suppressed (ICAM1, EZR, MAP2K2, and NOS3) gene expressions in metabolic syndrome
Tabur S, Oztuzcu S, Oguz E, Demiryürek S, Dagli H, Alasehirli B, Ozkaya M, Demiryürek AT
Endocrine 2016. 53(2):465-470
|
|
|
The Emerging Role of Arginase in Endothelial Dysfunction in Diabetes
Pernow J, Jung C
Curr Vasc Pharmacol 2016. 14(2):155-162
|
|
|
High glucose regulates LN expression in human liver sinusoidal endothelial cells through ROS/integrin αvβ3 pathway
Liu J, Quan J, Feng J, Zhang Q, Xu Y, Liu J, Huang W, Liu J, Tian L
Environ Toxicol Pharmacol 2016. 42:231-236
|
|
|
Post-transcriptional down regulation of ICAM-1 in feto-placental endothelium in GDM
Diaz-Perez FI, Hiden U, Gauster M, Lang I, Konya V, Heinemann A, Lögl J, Saffery R, Desoye G, Cvitic S
Cell Adh Migr 2016. 10(1-2):18-27
|
|
|
Resveratrol ameliorates high glucose-induced oxidative stress injury in human umbilical vein endothelial cells by activating AMPK
Zhou DY, Su Y, Gao P, Yang QH, Wang Z, Xu Q
Life Sci 2015. 136:94-9
|
|
|
Systemic Vascular Transduction by Capsid Mutant Adeno-Associated Virus After Intravenous Injection
Lipinski DM, Reid CA, Boye SL, Peterson JJ, Qi X, Boye SE, Boulton ME, Hauswirth WW
Hum Gene Ther 2015. 26(11):767-776
|
|
|
Lack of correlation between the optimal glycaemic control and coronary micro vascular dysfunction in patients with diabetes mellitus: a cross sectional study
Valenzuela-Garcia LF, Matsuzawa Y, Sara JD, Kwon TG, Lennon RJ, Lerman LO, Ruiz-Salmeron RJ, Lerman A
Cardiovasc Diabetol 2015. 14:106
|
|
|
Diabetes and cardiovascular disease: Epidemiology, biological mechanisms, treatment recommendations and future research
Leon BM, Maddox TM
World J Diabetes 2015. 6(13):1246-1258
|
|
|
Glycosylated hemoglobin and the risk of periprocedural myocardial infarction in non-diabetic patients
Verdoia M, Schaffer A, Barbieri L, Di Giovine G, Marino P, Suryapranata H, De Luca G, Novara Atherosclerosis Study Group (NAS)
J Diabetes Complications 2015. 29(4):517-522
|
|
|
Antioxidant Therapy: Is it your Gateway to Improved Cardiovascular Health?
Abid MR, Sellke FW
Pharm Analyt Acta 2015. 6:1
|
|
|
Alternative Medicine in Diabetes - Role of Angiogenesis, Oxidative Stress, and Chronic Inflammation
El-Refaei MF, Abduljawad SH, Alghamdi AH
Rev Diabet Stud 2014. 11(3-4):231-244
|
|
|
oxLDL induces injury and defenestration of human liver sinusoidal endothelial cells via LOX1
Zhang Q, Liu J, Liu J, Huang W, Tian L, Quan J, Wang Y, Niu R
J Mol Endocrinol 2014. 53(2):281-293
|
|
|
Oxidative stress, mitochondrial dysfunction and endoplasmic reticulum stress
Park Y
Bio Design 2014. 2(1):12-20
|
|
|
Combination therapy with losartan and L-carnitine protects against endothelial dysfunction of streptozotocin-induced diabetic rats
Sleem M, Taye A, El-Moselhy MA, Mangoura SA
Eur J Pharmacol 2014. 744:10-17
|
|
|
Renoprotective effect of atorvastatin on STZ-diabetic rats through attenuating kidney-associated dysmetabolism
Zhou S, Zhao P, Li Y, Deng T, Tian L, Li H
Eur J Pharmacol 2014. 740:9-14
|
|
|
Combination approaches to attenuate hemorrhagic transformation after tPA thrombolytic therapy in patients with poststroke hyperglycemia/diabetes
Fan X, Jiang Y, Yu Z, Yuan J, Sun X, Xiang S, Lo EH, Wang X
Adv Pharmacol 2014. 71:391-410
|
|
|
Research Advances on Mechanism of Cognitive Impairment in Type 2 Diabetes Mellitus
Chen F,Hu M,Du GT,Liu GJ,Hong H
Acta Neuropharmacol 2013. 3(3):27-33
|
|
|