Review
Rev Diabet Stud,
2014,
11(2):153-166 |
DOI 10.1900/RDS.2014.11.153 |
Effects of Dairy Protein and Fat on the Metabolic Syndrome and Type 2 Diabetes
Ann Bjørnshave, Kjeld Hermansen
Department of Endocrinology and Internal Medicine, Aarhus University Hospital, Tage-Hansens Gade 2, 8000 Aarhus C., Denmark
Address correspondence to: Ann Bjørnshave, e-mail: annovrga@rm.dk
Manuscript submitted April 7, 2014; resubmitted July 2, 2014; accepted July 9, 2014; published in print August 10, 2014.
Keywords: type 2 diabetes, metabolic syndrome, glucose metabolism, lipid metabolism, blood pressure, dairy fat
Abstract
The incidence of the metabolic syndrome (MetS) and type 2 diabetes (T2D) is increasing worldwide. Evidence supports a negative relationship between the consumption of dairy products and risk of MetS and T2D. Dairy proteins are known to have a directly beneficial effect on hypertension, dyslipidemia, and hyperglycemia, but a detailed understanding of the underlying mechanisms is missing. It has been confirmed by observations that the insulinotropic effect of dairy proteins is associated with the amino acid composition; in particular branched-chain amino acids (BCAA) seem to be of vital importance. Dairy protein-derived peptides may also contribute to the insulinotropic effect via dipeptidyl peptidase-4 (DPP-4) inhibitory activity, and may lower the blood pressure (BP). The lipid metabolism may be improved by whey protein (WP), which acts to reduce the postprandial triglyceride (TG) response. The effect of dairy fat is much more controversial because of the potentially harmful effect exerted by saturated fatty acid (SFA) on metabolic health. Recent observations suggest less adverse effects of SFA on metabolic health than previous assumed. However, little is known about dairy lipid fractions belonging to the groups of monounsaturated fatty acids (MUFA), polyunsaturated fatty acids (PUFA), and phospholipids (PL). Dairy fat seems to act differently depending on the dairy product and the composition of macronutrients in the meal. Therefore, for a better understanding of the mechanisms behind the dairy protein and fat effect on MetS, we suggest that more human studies should be carried out to clarify the interactions of dairy protein and fat with macronutrients in the meal and other dairy components, such as micronutrients and microorganisms from fermented products.
Abbreviations: ACE - angiotensin-converting enzyme; BCAA - branched-chain amino acids; BP - blood pressure; C10:0 - 10 carbons atom length; CVD - cardiovascular disease; DBP - diastolic blood pressure; DPP-4 - dipeptidyl peptidase-4; FFA - free fatty acids; GIP - glucose-dependent insulinotropic polypeptide; GLP-1 - glucagon-like peptide 1; HDL - high-density lipoprotein; HOMA-IR - homeostasis model assessment of insulin resistance; iAUC - incremental area under the curve; Lactopeptide C12 - Phe-Phe-Val-Ala-Pro-Phe-Pro-Glu-Val-Phe-Gly-Lys; LDL - low-density lipoprotein; LPL - lipoprotein lipase; MAP - mean arterial pressure; MetS - metabolic syndrome; mTOR - mammalian target of rapamycin; MUFA - monounsaturated fatty acids; NO - nitric oxide; PL - phospholipids; PUFA - polyunsaturated fatty acids; SBP - systolic blood pressure; SFA - saturated fatty acids; T2D - type 2 diabetes; TG - triglyceride; VLDL - very low-density lipoprotein; WP - whey protein; WPH - whey protein hydrolysate; WPI - whey protein isolate
1. Introduction
The incidence of metabolic syndrome (MetS) and type 2 diabetes (T2D) is increasing world-wide. MetS is characterized by a combination of metabolic characteristics, including abdominal obesity, dyslipidemia, impaired glucose homeostasis (impaired glucose tolerance or impaired fasting glycemia), and hypertension [1]. Furthermore, individuals with MetS are often affected by low-grade inflammation. These risk factors contribute to a 2-fold increased risk of developing cardiovascular disease (CVD) [2] and a 5-fold risk of developing T2D [3].
Meta-analyses and systematic reviews show an inverse association between consumption of dairy products and risk of MetS [4, 5] and T2D [6-8], respectively. Individual risk markers such as low-grade inflammation [9] and hypertension are also inversely associated with dairy consumption [10]. Some findings refer to dairy products in general [5, 7], others to specific low-fat dairy products [6, 8, 11]. Consumption of cheese and a mixture of fermented dairy product are also inversely associated with diabetes incidence [12, 13]. Recently, a review concluded that even a small rise from 2-3 to 4-5 daily servings of dairy products adds to the beneficial effect [14]. This is in agreement with a study suggesting that dairy intake reduces the risk of T2D in a dose-dependent manner [7].
The main ingredient of dairy products is milk. Milk consists of 87% water, 3.5% protein, 3.4-4.0% fat, and 4.9% lactose. Dairy protein and fat are complex constituents and can be fractionated at many levels. Results from studies to-date indicate that the beneficial effects of different fractions of dairy protein and fat on metabolic disturbances such as MetS and T2D remain inconclusive. It is well established that consumption of dairy products reduces the risk of developing MetS [4, 5] and T2D [6-8]. Therefore, it is important to clarify how single components in the complex dairy food matrix affect MetS and T2D risk factors. This would help us to evaluate the compounds' therapeutic potential in combating T2D and CVD. Therefore, the aim of the present review is to examine the existing evidence on the effects of dairy constituents on glucose metabolism, lipid metabolism, and blood pressure (BP), with specific focus on the following components:
1. Dairy proteins, including whey protein (WP) and casein
2. Fat including saturated fatty acids (SFA), monounsaturated fatty acids (MUFA), polyunsaturated fatty acids (PUFA), and phospholipids (PL)
Furthermore, the review aims to clarify potential mechanisms of dairy protein and fat on the metabolic risk factors.
2. Consideration of the relevant literature
Studies appropriate for inclusion in this review were identified by searching nine electronic databases (bibliotek.dk, SveMed+, Medline, PubMed, Embase, The Cochrane Library, Cinahl, Web of Science, and Scopus) for relevant reviews and meta-analyses examining the effects of dairy protein and dairy fat on human individuals with MetS and T2D up to 21th of February 2014. Supplementary evidence from recent research publications, independent of species, were covered by including studies on dairy protein and fat, in relation to MetS and T2D, during the period 2004-2014. The following search terms were included: diabetes mellitus type 2, insulin, blood glucose, metabolic syndrome, hypertension, dyslipidemia, dairy protein, milk protein, whey protein, casein, dairy fat.
3. Milk and dairy proteins
Milk contains two protein fractions, namely casein and WP, representing 2.8% and 0.7% of the total weight of milk, respectively. Fractions are primarily separated by acid precipitation in the cheese-making process [15].
Over the past 20 years, investigation on dairy protein has increased. Today we know that dairy protein has a direct positive effect on hypertension, dyslipidemia, and hyperglycemia. Dairy protein may also improve metabolic health indirectly by increasing lean body mass and reducing obesity [16, 17, 18]. The beneficial effects are primarily linked to WP. There is no apparent difference between the effects of different dairy proteins on satiety and hunger, stimulating hormones, and body weight [19]. Furthermore, it is unknown whether dairy protein outperforms other dietary protein sources in relation to metabolic health.
4. Whey protein
The WP fraction is a heterogeneous, polymorphic group of proteins, dominated by β-lactoglobulin, α-lactalbumin, and glycomacropeptide [15]. Commercially, WP occurs in three different condensed forms: WP concentrate (WPC), WP isolate (WPI), and WP hydrolysate (WPH). WPC has 30-85% protein, whereas WPI has >90% protein [20]. WPI contains little or no lactose or fat, which is not separated from WPC. WPH is a peptide product derived from WP through a process involving enzymatic hydrolysis or microbial fermentation[21]. The absorption rate of WP is dependent on the degree of hydrolysis and thereby the size of the available peptides. Consumption of WPH results in a more rapid increase of plasma amino acids compared with intact WP [22].
4.1 Glucose metabolism
The consumption of WP is known to acutely reduce the postprandial glucose response in both healthy [23-26] and T2D subjects [27]. WP also improves the insulin response in lean individuals [24, 28] and diabetics [27]. In healthy Japanese subjects, the consumption of WPH induces greater insulin stimulation than intact WP [22]. The consumption of WP 30 minutes prior to a carbohydrate-rich meal stimulates insulin and incretin secretion more than WP as part of a mixed meal in T2D subjects [29]. These results indicate that WP acutely improves glycemic control by stimulating insulin secretion, and reduces plasma glucose levels, an effect that may be enhanced by consumption of WP prior to meal. Thus, in healthy adults, WP contributes to an insulin-independent reduction of blood glucose concentration [26].
A couple of studies have investigated the impact of chronic consumption of WP on glucose and insulin homeostasis. In healthy animals, insulin sensitivity improved after consumption of WP within a period of 6 weeks, as indicated by reduced plasma insulin concentrations [30]. Continued consumption (12 weeks) of 55 g/day WP in obese individuals improved fasting insulin levels and homeostasis model assessment of insulin resistance (HOMA-IR) scores compared with control in overweight and obese subjects [31]. These results point to a beneficial effect of WP by way of continued ingestion, but further studies on MetS and T2D subjects are needed to confirm this. The results are summarized in Table 1.
Table
1.
Effects of milk proteins on glucose metabolism in human subjects |
|
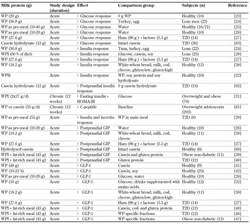 |
 |
Legend:
↑: significant increase. ↓: significant decrease. →: unchanged or insignificant effect compared with the comparison group. GIP: glucose-dependent insulinotropic polypeptide, GLP-1: glucagon-like peptide 1, HOMA-IR: homeostasis model assessment of insulin resistance, T2D: type 2 diabetes, WP: whey protein, WPH: whey protein hydrolysate, WPI: whey protein isolate. |
|
The insulinotropic effect of WP is probably linked to the high content of specific amino acids. A mixture of leucine, isoleucine, valine, threonine, and lysine can mimic glycemic and insulinemic responses similar to those generated by WP [32]. The effect of amino acid-mediated insulin secretion from pancreatic β-cells is substantial, but depends on the kind and composition of amino acids included in the mixture. Liu et al. demonstrated that arginine, lysine, alanine, proline, and glutamine stimulate insulin secretion from isolated mouse islets and clonal INS-1E β-cells in a dose- and glucose-dependent manner [33]. Leucine, isoleucine, and valine belong to the group of branched-chain amino acids (BCAA). BCAA are metabolized by branched-chain aminotransferase and branched-chain α-keto acid dehydrogenase. The liver has little aminotransferase activity, and therefore BCAA are primarily delivered to the systemic circulation and metabolized in the skeletal muscle. Additionally, BCAA (especially leucine) activate both the mammalian rapamycin (mTOR) pathway and protein synthesis leading to an increased hormone (e.g. insulin, GIP) expression and secretion [34, 35]. mTOR might also potentially improve insulin sensitivity by activating IRS-1 through phosphorylation [36].
Another possible explanation for the insulinotropic effect of WP is through activation of the incretin system. The incretin system involves glucose-dependent insulinotropic polypeptide (GIP) and glucagon-like peptide 1 (GLP-1). The hormones are released from the intestine in response to a meal, and subsequently further stimulate insulin secretion [37]. WP consumption increases postprandial GIP response in both healthy individuals [26, 38] and T2D patients [27] more than other dairy or protein sources or water. However, the GIP response cannot be mimicked by the supply of specific amino acids that are abundant in WP (BCAA, threonine and lysine) [32]. This indicates that the effect of GIP is not mediated via amino acids per se. Interestingly, in the initial postprandial period, the GIP response is lower after ingestion of WP in combination with a fat-rich diet than after ingestion of other dietary protein sources in both obese non-diabetic [39] and T2D subjects [40].
The effect of WP on GLP-1 is ambiguous. Two studies, comparing WP with soy and casein, report a rise in GLP-1 levels in healthy subjects [41, 42]. WP consumed as a pre-meal resulted also in a higher GLP-1 concentration in healthy subjects [26]. This may be related to dipeptidyl peptidase-4 (DDP-4) inhibitory activity of dairy protein, as shown in an animal study [43]. DPP-4 is an enzyme, which plays a major role in glucose metabolism by degrading GLP-1; hydrolytic products of α-lactalbumin and β-lactoglubulin possess DDP-4 inhibitory activity [44, 45]. Other studies that investigated the GLP-1-stimulating effect of WP in healthy [32, 38] and T2D subjects [27, 40, 46] did not detect any changes in the GLP-1 responses when expressed as incremental area under the curve (iAUC). Also, no difference was found in obese, non-diabetic subjects between WPI and WP-specific fractions (WPH and WP enhanced with α-lactalbumin and caseinoglycomacropeptide, respectively) [47]. The incretin results are summarized in Table 1.
4.2 Lipid metabolism
The effect of WP on lipid metabolism has been studied in a few acute and chronic setups. In acute studies, postprandial triglyceride (TG) responses measured as iAUC decreased significantly in both obese non-diabetic subjects [39] and T2D subjects [40] after consumption of WPI in comparison with other dietary protein sources (cod, casein or gluten) or glucose. In contrast, no difference in postprandial TG responses was observed when the acute effects of WPI and WP-specific fractions in obese, non-diabetics [47] and T2D subjects [46] were compared. Furthermore, studies comparing WP to other dietary protein sources found a suppression of the response to free fatty acids (FFA) [39, 40]. Interestingly, this WPH caused less postprandial suppression of FFA than WPI and other WP-specific fractions [47]. Intervention with WPI (54 g/day) in overweight and obese individuals reduced fasting TG by 22% after 6 weeks and by further 22% after 12 weeks compared with glucose intervention over the same time frame. Furthermore, WPI reduced total cholesterol and LDL cholesterol more than casein and glucose [31]. However, 12-week intervention with WP (50 g/day) did not affect fasting lipid parameters in moderate obese subjects [48]. Table 2 summarizes the effects of WP on lipid metabolism.
Table
2.
Effects of milk proteins on lipid metabolism in human subjects |
|
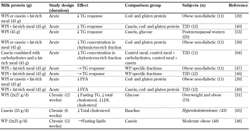 |
 |
Legend:
↑: significant increase. ↓: significant decrease. →: unchanged or insignificant effect compared with the comparison group. FFA: free fatty acids, T2D: type 2 diabetes, TG: triglycerides, WP: whey protein, WPI: whey protein isolate. |
|
Contrary to the results from chronic studies, findings from six acute studies noted no changes in other parameters related to lipid metabolism such as total cholesterol, low-density lipoprotein (LDL) cholesterol, or high-density lipoprotein (HDL) cholesterol. This may indicate that WP has an outstanding acute postprandial effect on the TG response compared to other dietary protein sources and glucose, and that the effect can either be attributed to WPI or a single WP fraction alone. The reason for this difference is unknown. Postprandial TG concentration is affected by many factors, e.g., intestinal chylomicron secretion, hepatic very low-density lipoprotein (VLDL) secretion, conversion of TG-rich lipoproteins to TG-depleted lipoproteins, and tissue uptake of TG-depleted lipoproteins [49]. Thus, WP consumption may result in a lower production of chylomicrons, which is consistent with the more pronounced FFA suppression. Lipoprotein lipase (LPL) is a key enzyme in the hydrolysis of TG in chylomicrons and VLDL. The insulinotropic effect of WP may enhance LPL activity, and hence accelerate chylomicron clearance. In the chronic state, WP is also proposed to affect intestinal lipid absorption and/or excretion and/or de novo cholesterol biogenesis in the liver [31].
Amino acids with high availability such as BCAA and essential amino acids contained in WP may possibly influence lipid metabolism. Lysine is involved in the metabolism of long-chain fatty acids, which are essential for the endogenous synthesis of carnitine. Lysine deficiency reduces carnitine concentrations in the body and increases lipid accumulations. Supplementation of lysine reduces the level of TG in tissues [50]. However, a 12-week intervention with lysine did not change the lipid profile in men with hypertriglyceridemia [50]. Leucine and alanine have also been linked to beneficial metabolic effects, such as reduced body fat accumulation [36, 51]. However, some investigations have revealed that the beneficial effects might be due to the supply of nitrogen rather than leucine itself. Thus, to better understand the mechanism by which WP affects lipid metabolism in general and TG responses in particular requires more investigation into the effect of single amino acids.
4.3 Blood pressure
Recent studies investigating the antihypertensive effect of milk protein and peptides are inconclusive, despite growing evidence that supports the hypothesis that milk proteins and peptides have beneficial effects on vascular health [52]. Only two studies in humans have compared the effects of WP and casein on BP. They found that WPI (45 g/day) compared to casein and glucose did not acutely change systolic blood pressure (SBP) or diastolic blood pressure (DBP) in postmenopausal women [53]. However, consumption of WP and casein 2 x 27 g/day during a 12-week period was found to reduce DBP in non-hypertensive subjects compared to glucose [54].
BP is controlled and regulated by different biochemical mechanisms. The renin-angiotensin system is a central pathway causing vasodilation and consequently decreased BP by inhibition of the angiotensin-converting enzyme (ACE). Peptides derived from casein are known to have an antihypertensive effect via inhibition of the ACE system. However, consumption of milk drinks supplemented with peptides from WP did not reduce BP in hypertensive subjects [55]. Besides the effect on the ACE system, the vasodilator nitric oxide (NO) also plays a major role in BP regulation. A whey-derived peptide (NOP-47) has been found to improve the arterial vasodilation in healthy women and men after 2 weeks intervention (5 g/day) [56]. NOP-47 is suggested to increase NO synthesis in the endothelium. Nevertheless, Ballard et al. observed that NOP-47 acutely (within 2 hours) increased brachial artery flow-mediated dilation in overweight persons, without causing inhibition of ACE activity or detectable changes in circulating endothelium-derived factors including plasma NO, prostacyclin, and endothelium-1 [57].
Several studies have investigated the influence of intact WP or WPH on BP in young people with elevated SBP and DBP. Consumption of WP and WPH (28 g/day) during a 6-week period reduced SBP, DBP, and mean arterial pressure (MAP) compared with baseline, with no significant difference between the two groups of young individuals consuming 28 g per day of either hydrolyzed or non-hydrolyzed whey protein [58]. Likewise, SBP and DBP were reduced in hypertensive subjects after 6 weeks of ingestion of WP-derived peptides (20 g/day) [59]. Therefore, whey-derived peptides may have a potential to reduce both SBP and DBP, however more studies are needed to identify the activating peptides and their mechanism of action. The results are summarized in Table 3.
Table
3.
Effects of milk proteins on blood pressure in human subjects |
|
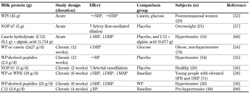 |
 |
Legend:
↑: significant increase. ↓: significant decrease. →: unchanged or insignificant effect compared with the comparison group. BP: blood pressure, C12: lactopeptide C12 (Phe-Phe-Val-Ala-Pro-Phe-Pro-Glu-Val-Phe-Gly-Lys), DBP: diastolic blood pressure, MAP: mean arterial pressure, NOP-47: whey-derived peptide, SBP: systolic blood pressure, T2D: type 2 diabetes, WP: whey protein, WPH: whey protein hydrolysate, WPI: whey protein isolate. |
|
5. Casein
Casein is the milk protein fraction that precipitates upon acidification. Consequently, casein as opposed to WP coagulates in the acidic environment in the stomach. This coagulation delays the gastric emptying and reduces the postprandial increase in plasma amino acids. Casein is known to be more slowly digested than WP, and the different digestion rates of the proteins may affect the insulin response. According to Bendtsen et al. this may result in a more pronounced satiating effect of casein over time [19]. Consumption of hydrolyzed casein results in a more rapid increase in plasma amino acid concentrations compared with intact casein [60]. The main components of the casein fraction are αs1-, αs2-, β-, and κ-casein.
5.1 Glucose metabolism
The effect of casein on glucose metabolism is not as well studied as the effect of WP (Table 1). Casein is often used as a protein benchmark in studies on WP. Acutely both WP and casein show insulinotropic properties in healthy subjects, with the WP fraction being a more potent insulin secretagogue than casein [38]. Continued consumption of 35 g casein/day (over 12 weeks) increased the concentration of C-peptide in the plasma of overweight adolescents [61]. The slow absorption of casein can be regulated by hydrolysis of the casein fraction. This ensures faster digestion and thereby quicker availability of BCAA, essential amino acids, and total plasma amino acids in the circulation [60]. Hydrolyzed casein in doses higher than 12 g/day enhance the carbohydrate-induced insulin and glucose responses compared to lower doses [62] and to intact casein [63] in T2D subjects. Interestingly, the induction from hydrolyzed casein was independent of the leucine content. The incretin hormones GIP and GLP-1 are proposed only to play a minor role in the insulinotropic effect of casein in T2D subjects [64]. However, hydrolyzed casein stimulates the release of GIP more substantially than intact casein, whereas the GLP-1 response is independent of protein fractionation [60].
5.2 Lipid metabolism
The consumption of casein in combination with carbohydrates and a fat-rich meal acutely suppresses the TG response in the chylomicron-rich fraction in T2D [64]. This is in agreement with observations in non-diabetic subjects, where casein and WP have similar suppressive effects on postprandial TG levels and TG concentrations in the chylomicron-rich fraction after a fat-rich meal [39]. An enhanced TG response is a common finding in MetS and T2D subjects as a part of postprandial dyslipidemia, which is due to insulin resistance. Therefore, the effect of casein is beneficial for the lipid metabolism.
In agreement with this finding, Holmer-Jensen et al. observed a positive correlation between FFA and plasma levels of TG after WP and casein consumption, primarily as a result of more pronounced FFA suppression [39]. This indicates that both WP and casein are able to reduce lipotoxicity. Other studies have found that WP is more efficient than casein in reducing the postprandial TG response in overweight post-menopausal women [53] and T2D subjects [40]. A six-week intake of casein reduces plasma total cholesterol in hypercholesterolemic adults compared with baseline, but the reduction was mainly caused by a decrease in HDL cholesterol [65]. The results are summarized in Table 2.
5.3 Blood pressure
Specific peptides derived from casein have antihypertensive effects by inhibiting the ACE system. Among those are the lactotripeptides Val-Pro-Pro and Ile-Pro-Pro. Two meta-analyses of randomized controlled trials report that the lactotripeptides reduce BP both in European and Asian subjects [66, 67]. Another lactopeptide C12 (Phe-Phe-Val-Ala-Pro-Phe-Pro-Glu-Val-Phe-Gly-Lys) is also able to reduce BP, by a mechanisms of ACE inhibition. Townsend et al. report that in hypertensive subjects lactopeptide C12 (100 mg) in combination with alginic acid (1754 mg) reduce both SBP (9.2 mmHg) and DBP (6.0 mmHg) 6 hours after oral administration [68]. Administration of lactopeptide C12 in a higher dose (3.8 g) reduces SBP and DBP in pre-hypertensive subjects [69]. The continued effect (12 weeks) of intact casein (54 g/day) was only tested in one study by Pal and Ellis [54], where casein and WPI demonstrate similar suppressive effects on DBP in overweight individuals. Therefore, more randomized, controlled intervention studies are needed to disentangle the potential benefits of both casein and WP on vascular health in general and BP in particular. Table 3 summarizes the effect of milk proteins on blood pressure.
6. Dairy fat
The results from only a few randomized clinical trials are published on the effect of dairy fat as a specific macronutrient in relation to MetS and T2D. However, there is a considerable amount of literature dealing with observational evidence. In recent years, consumption of dairy fat and its role in MetS and T2D has been extensively discussed. Dairy fat has been proposed as unlikely to independently protect against diabetes due to the association between low-fat and reduced risk of diabetes [70]. Although previous data indicate that dairy fat and especially high-fat dairy food contribute to obesity and cardiometabolic risk, a recent review rejects this hypothesis [71]. Likewise, there is no convincing evidence between high-fat dairy consumption and risk of T2D [71]. Consequently, the present evidence concludes that dairy fat consumption is not typically associated with an increased risk of weight gain, CVD, and T2D. Furthermore, SFA may be less adverse than previously thought [72, 73]. SFA comprises a range of distinct SFAs, which may affect the metabolism differentially.
Milk fat occurs as globules emulsified in the aqueous phase of milk. The globule is a complex substance composed of different layers, where the outermost protect against coalescing and stabilizing of the emulsion. The large surface of the globule makes it sensitive to lipolytic enzymes, while passing through the gastrointestinal tract.
The composition of the lipid fraction in milk is dominated by TG (95-96%). The remaining percentage is composed of phospholipids (PL), cholesterol, 1,2-diacylglycerol, FFA, monoacylglycerol, cholesteryl ester, and hydrocarbons [74]. The fatty acid composition is dominated by SFA (70%) followed by MUFA (25%) and PUFA (2.3%) [75]. Milk is characterized by a relatively high content of low molecular weight fatty acids, with approximately 400 different fatty acids, where butyric acid (C4:0) is the primary constituent. About 15 of these fatty acids are present in >1% and the others are present only in trace amounts. Furthermore, milk contains odd numbered fatty acids, e.g. pentadecanoic acid (C15:0) and margaric acid (C17:0).
Fatty acids originate from two sources: directly from peripheral circulation or from de novo synthesis. Mammary de novo synthesis accounts for all C4:0-C12:0, most of myristic acid (C14:0), and typically about half of palmitic acid (C16:0). Microorganisms in the rumen are able to synthesize C15:0 and C17:0 plus hydrogenate linoleic acid (C18:2) to yield oleic acid (C18:1) and stearic acid (C18:0) together with the formation of conjugated linoleic acids (CLA) [76]. C18:0 or longer are derived from circulating plasma lipids. The composition of milk lipids varies during seasons, and depends on genetic and environmental factors [74].
6.1 Saturated fat
C14:0, C16:0, and C18:0 are the most abundant SFAs. The remaining SFAs appear in <5% of the total [74]. Reducing the intake of SFA is recommended to avoid the negative effects on LDL-cholesterol, inflammation, and insulin sensitivity [77, 78]. However, Kuipers et al. suggest that reduction of carbohydrates with a high glycemic index may be more efficient in the prevention of CVD induced by low-grade inflammation [79]. Furthermore, substitution of starch with SFA is found to improve glycemic control in T2D subjects [80]. Besides the interaction with other macronutrients, SFA may also modulate the susceptibility to impaired insulin sensitivity, and may cause MetS in genetically disposed individuals [81]. Toll-like receptor 4 (TLR-4) is likely to be involved in the effects of SFA. SFA may activate TLR-4 signaling, thus increasing inflammatory cytokine productions and insulin resistance [82].
As mentioned above, SFA is referred to as dietary SFA in general. Literature on dairy-derived SFA is limited. Butter is often referred to as a source of SFA due to its high SFA content. In mixed meals, butter contributes more to the incretin effect by increasing insulin secretion than protein represented by dried meat [83]. In spite of the capability to stimulate insulin secretion, dairy SFA may impair insulin signaling through stimulation of low-grade inflammation, thus initiating insulin resistance [84]. C16:0 inhibits the activation of insulin signaling molecules (IRS-1, Akt, PI3-K), and causes insulin resistance [85]. C16:0 may also inhibit insulin signaling through sphingolipid synthesis, whereby C16:0 may serve as a precursor [86]. Substitution of butter with sunflower oil, a rich source in PUFA, induces a significant reduction in inflammatory markers in overweight men [87]. This observation points to adverse effects of dairy SFA on low-grade inflammation.
Synthetic C6:0-C10:0 (medium-chain fatty acids) have beneficial effects on metabolic health and improve insulin sensitivity. Under certain in vitro conditions, they are proinflammatory, but in vivo they may reduce intestinal injury and protect from hepatotoxicity [88]. C6:0-C10:0 fatty acids from dietary sources are found to be incorporated in adipose tissue TG and potentially affect adipose tissue and systemic functions. The SFAs, C12:0-C16:0, increase LDL and HDL cholesterol, and thereby have an unfavorable effect on the lipid profile [89]. On the other hand, specifically designed butter with 20% lower content of C12:0-C16:0 does not change inflammatory markers in healthy subjects [90]. Substituting butter for sunflower oil decreases the late phase of postprandial lipidemia in overweight men, which may be elicited via a slower absorption of SFA and a lower clearance rate of C18:0 and C16:0 [87].
The effect of SFA on the metabolism may also be affected by the remaining components in the given product. Hjerpsted et al. showed that cheese and butter with the same fat content and composition do not affect the lipid metabolism in the same way [91]. Cheese lowers LDL and HDL cholesterol concentrations after 6 weeks intervention. The explanation of this observation may be associated with other components than SFA, but the mechanism is not known. The authors propose potential effects of calcium, fermentation levels, or the protein content in cheese. Another explanation may be related to the mechanical handling of milk during processing which may cause a restructuring of elements contained in the milk fat globule.
6.2 Monounsaturated fat
Palmitoleic acid (cis and trans C16:1) and oleic acid (C18:1) are the MUFAs with quantitative significance in the dairy lipid fraction. They represent 1-2% and 20-30% of the total fatty acid composition in milk fat, respectively [74]. In the cohort of the Multi-Ethnic Study of Atherosclerosis, circulating levels of trans C16:1n-7 are associated with higher LDL cholesterol [92]. Conversely, trans C16:1n-7 is also associated with reduced TG, fasting insulin, and incidence of diabetes [92]. This is in agreement with previous results from the same group determined in the cohort of the Cardiovascular Health Study [93]. These results are in accordance with Kratz et al. who suggest trans C16:1 to be beneficial for obesity and metabolic health [71].
C18:1 is suggested as a dietary element reducing the incidence of metabolic diseases [78]. In mice, C18:1 augments the insulin response by 1.5-fold, without stimulating insulin secretion [94]. Milk supplemented with C18:1 and in combination with folic acid and vitamins A, B6, D, and E reduce total, LDL, and HDL cholesterol in subjects with MetS [95] and moderate CVD risk [96]. The three milk supplements applied were not isocaloric. Therefore it is hard to know the absolute effect of C18:1. There is possibly a synergistic effect between C18:1, milk, and the added supplements.
6.3 Polyunsaturated fat
CLA (C18:2) is one of the most studied fatty acids in a dietary context. It represents 1-3% of the total fatty acid composition in milk [74]. The primary CLA isomers in dairy fat is cis-9, trans 11 CLA (70-90%); the second most frequent CLA is trans 10, cis-12. Despite CLA unlikely affect the risk factors associated with MetS and T2D in humans [71], the consumption of trans 10, cis-12 CLA isomer exerts a negative effect on metabolic risk factors by aggravating insulin resistance and increasing plasma glucose concentration in obese men [97]. Consumption of a mixture (4.2 g/day) of cis-9, trans 11 CLA and trans 10, cis-12 CLA for three months increased the inflammatory marker C-reactive protein compared to control [98]. However, naturally enriched cis-9, trans 11 CLA cheese reduces IL-6, IL-8, and TNF-α in healthy subjects after 10 weeks intervention [99]. Taken together, the results from studies on CLA isomers are ambiguous.
α-linolenic acid is another major PUFA in milk; it comprises 0.5-2% of the total fatty acid composition in milk [74]. Djousse et al. report that subjects consuming ≥2 servings of dairy products per day together with a high intake of linolenic acid have the lowest prevalence of hypertension [10]. However, no interaction between dairy and linolenic acid consumption was observed.
6.4 Phospholipids
Polar lipids in milk, such as phosphorylated lipids, are concentrated in milk fat globule membranes surrounding the lipid droplets that are secreted by the mammary gland. The milk fat globule membrane has a complex structure. The key function is to prevent coalescence of fat globules in milk [100]. Phospholipids (PL) in milk represent approximately 0.25 to 0.96% of milk fat, with variability in the content attributed to numerous factors including the feeding regime, period of lactation, and differences in the methods of extraction and analysis [100].
Sphingomyelin, one of the PL contained in milk, accounts for about 1% of dairy fat [74]. Sphingomyelin is bioactive through its metabolites ceramide, spingosine, and sphingosine 1-sphosphate. Studies in animal models suggest an inhibitory effect of milk-derived sphingolipids on cholesterol absorption [101].
Ohlsson et al. have investigated whether buttermilk enriched with polar milk lipids, primarily sphingomyelin, acutely affect postprandial lipidemia after a fat-rich meal in healthy men [102]. They found no significant difference in plasma TG concentrations, but it seems to increase HDL cholesterol when compared with control who did not receive the sphingolipids within the milk formulation. Recently, Keller et al. assessed the effects of lipamin M20 on plasma lipids [103]. Lipamin M20 is a milk phospholipid concentrate prepared from butter concentrate. It contains 52% protein, 5% lactose, 38% total fat, and 18.4% total phospholipid. In this open-label, cross-over study of lipamin M20, healthy women consumed low and high doses of milk phospholipids (PL) and a combination of PL and plant sterol over a test period of ten days. The low dose comprised approximately 3 g of milk PL daily (corresponding to approximately 16.2 g lipamin M20), the high dose comprised approximately 6 g of milk PL (corresponding to approximately 32.4 g lipamin M20), and the combination dose comprised approximately 6 g of milk PL combined with 2 g plant sterols. Intake of 32.4 g lipamin M20 provided approximately 16.8 g protein (milk fat globule membrane fraction not specified) and 6 g PL to the body. Total plasma PL, phosphatidylcholine, sphingomyelin, and phosphatidyl inositol concentrations did not differ from baseline values or between groups after the interventions with lipamin M20 [103]. Following intake of 16.2 g lipamin 20, the plasma level of phosphatidyl ethanolamine was higher than at baseline. Total and HDL cholesterol levels in plasma were significantly lower after daily intake of 16.2 g lipamin 20 compared with levels at baseline, while total and LDL cholesterol levels following intake of 32.4 g lipamin 20 were significantly higher than levels after intake of 16.2 g lipamin 20. Stool weight was higher following daily intake of 6 g milk PL compared with baseline [103]. Fecal pH was significantly lower following the intervention than at baseline, and fecal weight of PL (mg/g dry matter) increased.
Conway and colleagues assessed the effects of daily intake of two servings of buttermilk (prepared from powder, 45 g/day) on plasma lipids, sterols, C-reactive protein, and BP in a randomized, controlled, crossover study [104, 105]. The total daily intake of PL from the buttermilk and control beverages was 188 mg and 34 mg, respectively. Thirty-four adults completed the study and consumed buttermilk or the control beverage for consecutive periods of four weeks. Total cholesterol, TG, and BP were significantly lower after consumption of buttermilk than after consumption of control beverage. There were no changes in HDL cholesterol or body weight.
Human studies indicate that milk PL may have a regulating impact on the lipid metabolism. However, more studies are needed to determine the mechanism of PL.
7. Conclusions and perspectives
Taken together, dairy proteins have a high potential to improve metabolic disturbances in relation to MetS and T2D due to the amino acid composition. To identify the mechanisms underlying the protein-specific insulinotropic effects more studies are needed investigating specific amino acids and dairy protein-derived peptides. Likewise, we need to gain understanding of the beneficial properties on lipid metabolism in general and plasma TG in particular by testing the effects of specific amino acids and dairy protein-derived peptides. Interestingly, dairy proteins have a DPP-4 inhibitory potential, which possibly can improve glucose metabolism. This should be tested in humans.
Little is known about the specific dairy lipid fractions, not least the potential differences between short- and medium-chain SFA versus long-chain SFA and their effects on metabolic risk factors. Human studies are needed on specific fatty acids contained in SFA, MUFA, PUFA, and PL to determine their potential effects and underlying mechanisms. Moreover, we need studies to clarify the effects of dairy products on metabolic risk factors and the mechanisms by which dairy lipids act and if they possibly interact synergistically with other dietary components. A large part of our knowledge derives from observational studies that do not allow the identification of cause-effect relationship. Therefore, long-term, randomized, controlled human clinical trials are needed to clarify whether dairy products with various SFA compositions influence the metabolism differentially in MetS and T2D subjects.
It will also be of interest to study the impact of dairy proteins and fat on body composition and fat distribution (gynoid versus android distribution) as these measures play a key role in the risk of CVD. An important question is how dairy proteins and fat impact diurnal BP and pulse (i.e. the influence over 24 h). It seems that dairy proteins counteract inflammatory factors, but we need a more accurate picture of the effects of dairy components on both fasting and postprandial levels of inflammation, which are critical aspects in atherogenesis and the development of CVD. Apparently, low-fat-fermented dairy products have a positive influence on T2D risk [13]. However, we have little knowledge about the mechanisms of action. The beneficial effects of fermented dairy products on various risk factors may be related to probiotic bacteria, vitamin K2, and energy density. This needs to be explored in more detail.
In conclusion, the present evidence confirms that dairy proteins have an insulinotropic effect, but the underlying mechanism is not yet clear. The amino acid composition and specific amino acids like BCAA seem to be of particular importance. Dairy protein-derived peptides may also contribute to the insulinotropic effect via DPP-4 inhibitory activity and improvement of BP. Data indicate that in particular dairy fat has different effects depending on the dairy product and composition of macronutrients in the meal. To better understand the biological mechanisms of dairy proteins and fat on metabolic risk factors more human studies are needed; they should focus on the interactions of dairy proteins and fat with macronutrients in the meal and other dairy components such as micronutrients and microorganisms from fermented products. Also, there appears to be an impact of manufacturing methods of dairy products on their potential to act insulinotropic or antiatherogenic. This should be determined. Although not easy to achieve, these findings will provide a more clear knowledge of the bioactive mechanisms of dairy proteins and fat.
Disclosures: The authors report no conflict of interests.
Acknowledgments:
This work was funded by Danish Dairy Research Foundation, Future Food Innovation, Aarhus University, and 'Dairy lipids, proteins and the Metabolic Syndrome (DairyHealth)', a program financed by the The Danish Council for Strategic Research (no. 0603-00419B).
References
- Alberti KG, Zimmet P, Shaw J. Metabolic syndrome - a new world-wide definition. A consensus statement from the International Diabetes Federation. Diabet Med 2006. 23(5):469-480. [DOD] [CrossRef]
- Galassi A, Reynolds K, He J. Metabolic syndrome and risk of cardiovascular disease: a meta-analysis. Am J Med 2006. 119(10):812-819. [DOD] [CrossRef]
- Alberti KG, Eckel RH, Grundy SM, Zimmet PZ, Cleeman JI, Donato KA, Fruchart JC, James WP, Loria CM, Smith SC Jr. Harmonizing the metabolic syndrome: a joint interim statement of the International Diabetes Federation Task Force on Epidemiology and Prevention; National Heart, Lung, and Blood Institute; American Heart Association; World Heart Federation; International Atherosclerosis Society; and International Association for the Study of Obesity. Circulation 2009. 120(16):1640-1645. [DOD] [CrossRef]
- Crichton GE, Bryan J, Buckley J, Murphy KJ. Dairy consumption and metabolic syndrome: a systematic review of findings and methodological issues. Obes Rev 2011. 12(5):e190-e201. [DOD] [CrossRef]
- Pfeuffer M, Schrezenmeir J. Milk and the metabolic syndrome. Obes Rev 2007. 8(2):109-118. [DOD] [CrossRef]
- Tong X, Dong JY, Wu ZW, Li W, Qin LQ. Dairy consumption and risk of type 2 diabetes mellitus: a meta-analysis of cohort studies. Eur J Clin Nutr 2011. 65(9):1027-1031. [DOD] [CrossRef]
- Kalergis M, Leung Yinko SS, Nedelcu R. Dairy products and prevention of type 2 diabetes: implications for research and practice. Front Endocrinol (Lausanne) 2013. 4:90. [DOD]
- Aune D, Norat T, Romundstad P, Vatten LJ. Dairy products and the risk of type 2 diabetes: a systematic review and dose-response meta-analysis of cohort studies. Am J Clin Nutr 2013. 98(4):1066-1083. [DOD] [CrossRef]
- Panagiotakos DB, Pitsavos CH, Zampelas AD, Chrysohoou CA, Stefanadis CI. Dairy products consumption is associated with decreased levels of inflammatory markers related to cardiovascular disease in apparently healthy adults: the ATTICA study. J Am Coll Nutr 2010. 29(4):357-364. [DOD] [CrossRef]
- Djousse L, Pankow JS, Hunt SC, Heiss G, Province MA, Kabagambe EK, Ellison RC. Influence of saturated fat and linolenic acid on the association between intake of dairy products and blood pressure. Hypertension 2006. 48(2):335-341. [DOD] [CrossRef]
- Chrysant SG, Chrysant GS. An update on the cardiovascular pleiotropic effects of milk and milk products. J Clin Hypertens (Greenwich) 2013. 15(7):503-510. [DOD] [CrossRef]
- Sluijs I, Forouhi NG, Beulens JW, van der Schouw YT, Agnoli C, Arriola L, Balkau B, Barricarte A, Boeing H, Bueno-de-Mesquita HB, et al. The amount and type of dairy product intake and incident type 2 diabetes: results from the EPIC-InterAct Study. Am J Clin Nutr 2012. 96(2):382-390. [DOD] [CrossRef]
- O'Connor LM, Lentjes MA, Luben RN, Khaw KT, Wareham NJ, Forouhi NG. Dietary dairy product intake and incident type 2 diabetes: a prospective study using dietary data from a 7-day food diary. Diabetologia 2014. 57(5):909-917. [DOD] [CrossRef]
- Da Silva MS, Rudkowska I. Dairy products on metabolic health: current research and clinical implications. Maturitas 2014. 77(3):221-228. [DOD] [CrossRef]
- Smithers GW. Whey and whey proteins - from 'gutter-to-gold'. Int Dairy J 2008. 18(7):695-704. [DOD] [CrossRef]
- Sousa GT, Lira FS, Rosa JC, de Oliveira EP, Oyama LM, Santos RV, Pimentel GD. Dietary whey protein lessens several risk factors for metabolic diseases: a review. Lipids Health Dis 2012. 11:67. [DOD] [CrossRef]
- Pal S, Radavelli-Bagatini S. The effects of whey protein on cardiometabolic risk factors. Obes Rev 2013. 14(4):324-343. [DOD] [CrossRef]
- McGregor RA, Poppitt SD. Milk protein for improved metabolic health: a review of the evidence. Nutr Metab (Lond) 2013. 10(1):46. [DOD] [CrossRef]
- Bendtsen LQ, Lorenzen JK, Bendsen NT, Rasmussen C, Astrup A. Effect of dairy proteins on appetite, energy expenditure, body weight, and composition: a review of the evidence from controlled clinical trials. Adv Nutr 2013. 4(4):418-438. [DOD] [CrossRef]
- Foegeding EA, Luck P, Vardhanabhuti B. Whey Protein Products. In: Encyclopedia of Dairy Science. Elsevier, 2011. Chapter 873-878. [DOD]
- Ricci-Cabello I, Herrera MO, Artacho R. Possible role of milk-derived bioactive peptides in the treatment and prevention of metabolic syndrome. Nutr Rev 2012. 70(4):241-255. [DOD] [CrossRef]
- Morifuji M, Ishizaka M, Baba S, Fukuda K, Matsumoto H, Koga J, Kanegae M, Higuchi M. Comparison of different sources and degrees of hydrolysis of dietary protein: effect on plasma amino acids, dipeptides, and insulin responses in human subjects. J Agric Food Chem 2010. 58(15):8788-8797. [DOD] [CrossRef]
- Petersen BL, Ward LS, Bastian ED, Jenkins AL, Campbell J, Vuksan V. A whey protein supplement decreases post-prandial glycemia. Nutr J 2009. 8:47. [DOD] [CrossRef]
- Pal S, Ellis V. The acute effects of four protein meals on insulin, glucose, appetite and energy intake in lean men. Br J Nutr 2010. 104(8):1241-1248. [DOD] [CrossRef]
- Akhavan T, Luhovyy BL, Brown PH, Cho CE, Anderson GH. Effect of premeal consumption of whey protein and its hydrolysate on food intake and postmeal glycemia and insulin responses in young adults. Am J Clin Nutr 2010. 91(4):966-975. [DOD] [CrossRef]
- Akhavan T, Luhovyy BL, Panahi S, Kubant R, Brown PH, Anderson GH. Mechanism of action of pre-meal consumption of whey protein on glycemic control in young adults. J Nutr Biochem 2014. 25(1):36-43. [DOD] [CrossRef]
- Frid AH, Nilsson M, Holst JJ, Bjorck IM. Effect of whey on blood glucose and insulin responses to composite breakfast and lunch meals in type 2 diabetic subjects. Am J Clin Nutr 2005. 82(1):69-75. [DOD]
- Acheson KJ, Blondel-Lubrano A, Oguey-Araymon S, Beaumont M, Emady-Azar S, Ammon-Zufferey C, Monnard I, Pinaud S, Nielsen-Moennoz C, Bovetto L. Protein choices targeting thermogenesis and metabolism. Am J Clin Nutr 2011. 93(3):525-534. [DOD] [CrossRef]
- Ma J, Stevens JE, Cukier K, Maddox AF, Wishart JM, Jones KL, Clifton PM, Horowitz M, Rayner CK. Effects of a protein preload on gastric emptying, glycemia, and gut hormones after a carbohydrate meal in diet-controlled type 2 diabetes. Diabetes Care 2009. 32(9):1600-1602. [DOD] [CrossRef]
- Belobrajdic DP, McIntosh GH, Owens JA. A high-whey-protein diet reduces body weight gain and alters insulin sensitivity relative to red meat in wistar rats. J Nutr 2004. 134(6):1454-1458. [DOD]
- Pal S, Ellis V, Dhaliwal S. Effects of whey protein isolate on body composition, lipids, insulin and glucose in overweight and obese individuals. Br J Nutr 2010. 104(5):716-723. [DOD] [CrossRef]
- Nilsson M, Holst JJ, Bjorck IM. Metabolic effects of amino acid mixtures and whey protein in healthy subjects: studies using glucose-equivalent drinks. Am J Clin Nutr 2007. 85(4):996-1004. [DOD]
- Liu Z, Jeppesen PB, Gregersen S, Chen X, Hermansen K. Dose- and glucose-dependent ffects of amino acids on insulin secretion from isolated mouse islets and clonal INS-1E beta-cells. Rev Diabet Stud 2008. 5(4):232-244. [DOD] [CrossRef]
- Jakubowicz D, Froy O. Biochemical and metabolic mechanisms by which dietary whey protein may combat obesity and type 2 diabetes. J Nutr Biochem 2013. 24(1):1-5. [DOD] [CrossRef]
- Melnik BC. Leucine signaling in the pathogenesis of type 2 diabetes and obesity. World J Diabetes 2012. 3(3):38-53. [DOD] [CrossRef]
- Freudenberg A, Petzke KJ, Klaus S. Comparison of high-protein diets and leucine supplementation in the prevention of metabolic syndrome and related disorders in mice. J Nutr Biochem 2012. 23(11):1524-1530. [DOD] [CrossRef]
- Phillips LK, Prins JB. Update on incretin hormones. Ann N Y Acad Sci 2011. 1243:E55-E74. [DOD] [CrossRef]
- Nilsson M, Stenberg M, Frid AH, Holst JJ, Bjorck IM. Glycemia and insulinemia in healthy subjects after lactose-equivalent meals of milk and other food proteins: the role of plasma amino acids and incretins. Am J Clin Nutr 2004. 80(5):1246-1253. [DOD]
- Holmer-Jensen J, Mortensen LS, Astrup A, de VM, Holst JJ, Thomsen C, Hermansen K. Acute differential effects of dietary protein quality on postprandial lipemia in obese non-diabetic subjects. Nutr Res 2013. 33(1):34-40. [DOD] [CrossRef]
- Mortensen LS, Hartvigsen ML, Brader LJ, Astrup A, Schrezenmeir J, Holst JJ, Thomsen C, Hermansen K. Differential effects of protein quality on postprandial lipemia in response to a fat-rich meal in type 2 diabetes: comparison of whey, casein, gluten, and cod protein. Am J Clin Nutr 2009. 90(1):41-48. [DOD] [CrossRef]
- Hall WL, Millward DJ, Long SJ, Morgan LM. Casein and whey exert different effects on plasma amino acid profiles, gastrointestinal hormone secretion and appetite. Br J Nutr 2003. 89(2):239-248. [DOD] [CrossRef]
- Veldhorst MA, Nieuwenhuizen AG, Hochstenbach-Waelen A, van Vught AJ, Westerterp KR, Engelen MP, Brummer RJ, Deutz NE, Westerterp-Plantenga MS. Dose-dependent satiating effect of whey relative to casein or soy. Physiol Behav 2009. 96(4-5):675-682. [DOD] [CrossRef]
- Olivos DR, McGrath LE, Turner CA, Montaubin O, Mietlicki-Baase EG, Hayes MR. Intraduodenal milk protein concentrate augments the glycemic and food intake suppressive effects of DPP-IV inhibition. Am J Physiol Regul Integr Comp Physiol 2014. 306(3):R157-R163. [DOD] [CrossRef]
- Lacroix IM, Li-Chan EC. Inhibition of dipeptidyl peptidase (DPP)-IV and alpha-glucosidase activities by pepsin-treated whey proteins. J Agric Food Chem 2013. 61(31):7500-7506. [DOD] [CrossRef]
- Silveira ST, Martinez-Maqueda D, Recio I, Hernandez-Ledesma B. Dipeptidyl peptidase-IV inhibitory peptides generated by tryptic hydrolysis of a whey protein concentrate rich in beta-lactoglobulin. Food Chem 2013. 141(2):1072-1077. [DOD] [CrossRef]
- Mortensen LS, Holmer-Jensen J, Hartvigsen ML, Jensen VK, Astrup A, de VM, Holst JJ, Thomsen C, Hermansen K. Effects of different fractions of whey protein on postprandial lipid and hormone responses in type 2 diabetes. Eur J Clin Nutr 2012. 66(7):799-805. [DOD] [CrossRef]
- Holmer-Jensen J, Hartvigsen ML, Mortensen LS, Astrup A, de VM, Holst JJ, Thomsen C, Hermansen K. Acute differential effects of milk-derived dietary proteins on postprandial lipaemia in obese non-diabetic subjects. Eur J Clin Nutr 2012. 66(1):32-38. [DOD] [CrossRef]
- Claessens M, van Baak MA, Monsheimer S, Saris WH. The effect of a low-fat, high-protein or high-carbohydrate ad libitum diet on weight loss maintenance and metabolic risk factors. Int J Obes (Lond) 2009. 33(3):296-304. [DOD] [CrossRef]
- Karpe F. Postprandial lipoprotein metabolism and atherosclerosis. J Intern Med 1999. 246(4):341-355. [DOD] [CrossRef]
- Hlais S, Reslan DR, Sarieddine HK, Nasreddine L, Taan G, Azar S, Obeid OA. Effect of lysine, vitamin B(6), and carnitine supplementation on the lipid profile of male patients with hypertriglyceridemia: a 12-week, open-label, randomized, placebo-controlled trial. Clin Ther 2012. 34(8):1674-1682. [DOD] [CrossRef]
- Freudenberg A, Petzke KJ, Klaus S. Dietary L-leucine and L-alanine supplementation have similar acute effects in the prevention of high-fat diet-induced obesity. Amino Acids 2013. 44(2):519-528. [DOD] [CrossRef]
- Fekete AA, Givens DI, Lovegrove JA. The impact of milk proteins and peptides on blood pressure and vascular function: a review of evidence from human intervention studies. Nutr Res Rev 2013. 26(2):177-190. [DOD] [CrossRef]
- Pal S, Ellis V, Ho S. Acute effects of whey protein isolate on cardiovascular risk factors in overweight, post-menopausal women. Atherosclerosis 2010. 212(1):339-344. [DOD] [CrossRef]
- Pal S, Ellis V. The chronic effects of whey proteins on blood pressure, vascular function, and inflammatory markers in overweight individuals. Obesity (Silver Spring) 2010. 18(7):1354-1359. [DOD] [CrossRef]
- Lee YM, Skurk T, Hennig M, Hauner H. Effect of a milk drink supplemented with whey peptides on blood pressure in patients with mild hypertension. Eur J Nutr 2007. 46(1):21-27. [DOD] [CrossRef]
- Ballard KD, Bruno RS, Seip RL, Quann EE, Volk BM, Freidenreich DJ, Kawiecki DM, Kupchak BR, Chung MY, Kraemer WJ, et al. Acute ingestion of a novel whey-derived peptide improves vascular endothelial responses in healthy individuals: a randomized, placebo controlled trial. Nutr J 2009. 8:34. [DOD] [CrossRef]
- Ballard KD, Kupchak BR, Volk BM, Mah E, Shkreta A, Liptak C, Ptolemy AS, Kellogg MS, Bruno RS, Seip RL, et al. Acute effects of ingestion of a novel whey-derived extract on vascular endothelial function in overweight, middle-aged men and women. Br J Nutr 2013. 109(5):882-893. [DOD] [CrossRef]
- Fluegel SM, Shultz TD, Powers JR, Clark S, Barbosa-Leiker C, Wright BR, Freson TS, Fluegel HA, Minch JD, Schwarzkopf LK, et al. Whey beverages decrease blood pressure in prehypertensive and hypertensive young men and women. Int Dairy J 2010. 20(11):753-760. [DOD] [CrossRef]
- Pins JJ, Keenan JM. Effects of whey peptides on cardiovascular disease risk factors. J Clin Hypertens (Greenwich) 2006. 8(11):775-782. [DOD] [CrossRef]
- Calbet JA, Holst JJ. Gastric emptying, gastric secretion and enterogastrone response after administration of milk proteins or their peptide hydrolysates in humans. Eur J Nutr 2004. 43(3):127-139. [DOD] [CrossRef]
- Arnberg K, Molgaard C, Michaelsen KF, Jensen SM, Trolle E, Larnkjaer A. Skim milk, whey, and casein increase body weight and whey and casein increase the plasma C-peptide concentration in overweight adolescents. J Nutr 2012. 142(12):2083-2090. [DOD] [CrossRef]
- Jonker JT, Wijngaarden MA, Kloek J, Groeneveld Y, Gerhardt C, Brand R, Kies AK, Romijn JA, Smit JW. Effects of low doses of casein hydrolysate on post-challenge glucose and insulin levels. Eur J Intern Med 2011. 22(3):245-248. [DOD] [CrossRef]
- Geerts BF, van Dongen MG, Flameling B, Moerland MM, de Kam ML, Cohen AF, Romijn JA, Gerhardt CC, Kloek J, Burggraaf J. Hydrolyzed casein decreases postprandial glucose concentrations in T2DM patients irrespective of leucine content. J Diet Suppl 2011. 8(3):280-292. [DOD] [CrossRef]
- Brader L, Holm L, Mortensen L, Thomsen C, Astrup A, Holst JJ, de VM, Schrezenmeir J, Hermansen K. Acute effects of casein on postprandial lipemia and incretin responses in type 2 diabetic subjects. Nutr Metab Cardiovasc Dis 2010. 20(2):101-109. [DOD] [CrossRef]
- Weisse K, Brandsch C, Zernsdorf B, Nkengfack Nembongwe GS, Hofmann K, Eder K, Stangl GI. Lupin protein compared to casein lowers the LDL cholesterol:HDL cholesterol-ratio of hypercholesterolemic adults. Eur J Nutr 2010. 49(2):65-71. [DOD] [CrossRef]
- Turpeinen AM, Jarvenpaa S, Kautiainen H, Korpela R, Vapaatalo H. Antihypertensive effects of bioactive tripeptides-a random effects meta-analysis. Ann Med 2013. 45(1):51-56. [DOD] [CrossRef]
- Cicero AF, Aubin F, Azais-Braesco V, Borghi C. Do the lactotripeptides isoleucine-proline-proline and valine-proline-proline reduce systolic blood pressure in European subjects? A meta-analysis of randomized controlled trials. Am J Hypertens 2013. 26(3):442-449. [DOD] [CrossRef]
- Townsend RR, McFadden CB, Ford V, Cadee JA. A randomized, double-blind, placebo-controlled trial of casein protein hydrolysate (C12 peptide) in human essential hypertension. Am J Hypertens 2004. 17(11 Pt 1):1056-1058. [DOD] [CrossRef]
- Cadee JA, Chang CY, Chen CW, Huang CN, Chen SL, Wang CK. Bovine casein hydrolysate (c12 Peptide) reduces blood pressure in prehypertensive subjects. Am J Hypertens 2007. 20(1):1-5. [DOD] [CrossRef]
- Riserus U, Willett WC, Hu FB. Dietary fats and prevention of type 2 diabetes. Prog Lipid Res 2009. 48(1):44-51. [DOD] [CrossRef]
- Kratz M, Baars T, Guyenet S. The relationship between high-fat dairy consumption and obesity, cardiovascular, and metabolic disease. Eur J Nutr 2013. 52(1):1-24. [DOD] [CrossRef]
- Givens DI. Milk in the diet: good or bad for vascular disease? Proc Nutr Soc 2012. 71(1):98-104. [DOD]
- Huth PJ, Park KM. Influence of dairy product and milk fat consumption on cardiovascular disease risk: a review of the evidence. Adv Nutr 2012. 3(3):266-285. [DOD] [CrossRef]
- Jensen RG. The composition of bovine milk lipids: January 1995 to December 2000. J Dairy Sci 2002. 85(2):295-350. [DOD] [CrossRef]
- Mansson HL. Fatty acids in bovine milk fat. Food Nutr Res 2008. 2008:52. [DOD]
- Belitz HD, Grosch W, Schieberle P. Milk and Dairy Products. In: Food Chemistry. 2008, Chapter 10, pp 498-545. [DOD]
- Mason P, Porter SC, Berry SE, Stillman P, Steele C, Kirby A, Griffin BA, Minihane AM. Saturated fatty acid consumption: outlining the scale of the problem and assessing the solutions. Nutr Bull 2009. 34(1):74-84. [DOD] [CrossRef]
- Kennedy A, Martinez K, Chuang CC, LaPoint K, McIntosh M. Saturated fatty acid-mediated inflammation and insulin resistance in adipose tissue: mechanisms of action and implications. J Nutr 2009. 139(1):1-4. [DOD] [CrossRef]
- Kuipers RS, de Graaf DJ, Luxwolda MF, Muskiet MH, Dijck-Brouwer DA, Muskiet FA. Saturated fat, carbohydrates and cardiovascular disease. Neth J Med 2011. 69(9):372-378. [DOD]
- Hays JH, Gorman RT, Shakir KM. Results of use of metformin and replacement of starch with saturated fat in diets of patients with type 2 diabetes. Endocr Pract 2002. 8(3):177-183. [DOD] [CrossRef]
- Phillips CM, Goumidi L, Bertrais S, Field MR, McManus R, Hercberg S, Lairon D, Planells R, Roche HM. Dietary saturated fat, gender and genetic variation at the TCF7L2 locus predict the development of metabolic syndrome. J Nutr Biochem 2012. 23(3):239-244. [DOD] [CrossRef]
- Cuda C, Badawi A, Karmali M, El-Sohemy A. Polymorphisms in Toll-like receptor 4 are associated with factors of the metabolic syndrome and modify the association between dietary saturated fat and fasting high-density lipoprotein cholesterol. Metabolism 2011. 60(8):1131-1135. [DOD] [CrossRef]
- Carrel G, Egli L, Tran C, Schneiter P, Giusti V, D'Alessio D, Tappy L. Contributions of fat and protein to the incretin effect of a mixed meal. Am J Clin Nutr 2011. 94(4):997-1003. [DOD] [CrossRef]
- Funaki M. Saturated fatty acids and insulin resistance. J Med Invest 2009. 56(3-4):88-92. [DOD] [CrossRef]
- German JB, Gibson RA, Krauss RM, Nestel P, Lamarche B, van Staveren WA, Steijns JM, de Groot LC, Lock AL, Destaillats F. A reappraisal of the impact of dairy foods and milk fat on cardiovascular disease risk. Eur J Nutr 2009. 48(4):191-203. [DOD] [CrossRef]
- Deevska GM, Nikolova-Karakashian MN. The twists and turns of sphingolipid pathway in glucose regulation. Biochimie 2011. 93(1):32-38. [DOD] [CrossRef]
- Masson CJ, Mensink RP. Exchanging saturated fatty acids for (n-6) polyunsaturated fatty acids in a mixed meal may decrease postprandial lipemia and markers of inflammation and endothelial activity in overweight men. J Nutr 2011. 141(5):816-821. [DOD] [CrossRef]
- Marten B, Pfeuffer M, Schrezenmeir J. Medium-chain triglycerides. Int Dairy J 2006. 16(11):1374-1382. [DOD] [CrossRef]
- Mensink RP, Zock PL, Kester AD, Katan MB. Effects of dietary fatty acids and carbohydrates on the ratio of serum total to HDL cholesterol and on serum lipids and apolipoproteins: a meta-analysis of 60 controlled trials. Am J Clin Nutr 2003. 77(5):1146-1155. [DOD]
- Werner LB, Hellgren LI, Raff M, Jensen SK, Petersen RA, Drachmann T, Tholstrup T. Effects of butter from mountain-pasture grazing cows on risk markers of the metabolic syndrome compared with conventional Danish butter: a randomized controlled study. Lipids Health Dis 2013. 12:99. [DOD] [CrossRef]
- Hjerpsted J, Leedo E, Tholstrup T. Cheese intake in large amounts lowers LDL-cholesterol concentrations compared with butter intake of equal fat content. Am J Clin Nutr 2011. 94(6):1479-1484. [DOD] [CrossRef]
- Mozaffarian D, de Oliveira Otto MC, Lemaitre RN, Fretts AM, Hotamisligil G, Tsai MY, Siscovick DS, Nettleton JA. trans-Palmitoleic acid, other dairy fat biomarkers, and incident diabetes: the Multi-Ethnic Study of Atherosclerosis (MESA). Am J Clin Nutr 2013. 97(4):854-861. [DOD] [CrossRef]
- Mozaffarian D, Cao H, King IB, Lemaitre RN, Song X, Siscovick DS, Hotamisligil GS. Trans-palmitoleic acid, metabolic risk factors, and new-onset diabetes in U.S. adults: a cohort study. Ann Intern Med 2010. 153(12):790-799. [DOD] [CrossRef]
- Gunnarsson PT, Winzell MS, Deacon CF, Larsen MO, Jelic K, Carr RD, Ahren B. Glucose-induced incretin hormone release and inactivation are differently modulated by oral fat and protein in mice. Endocrinology 2006. 147(7):3173-3180. [DOD] [CrossRef]
- Fonolla J, Lopez-Huertas E, Machado FJ, Molina D, Alvarez I, Marmol E, Navas M, Palacin E, Garcia-Valls MJ, Remon B, et al. Milk enriched with "healthy fatty acids" improves cardiovascular risk markers and nutritional status in human volunteers. Nutrition 2009. 25(4):408-414. [DOD] [CrossRef]
- Benito P, Caballero J, Moreno J, Gutierrez-Alcantara C, Munoz C, Rojo G, Garcia S, Soriguer FC. Effects of milk enriched with omega-3 fatty acid, oleic acid and folic acid in patients with metabolic syndrome. Clin Nutr 2006. 25(4):581-587. [DOD] [CrossRef]
- Riserus U, Smedman A, Basu S, Vessby B. Metabolic effects of conjugated linoleic acid in humans: the Swedish experience. Am J Clin Nutr 2004. 79(6 Suppl):1146S-1148S. [DOD]
- Smedman A, Basu S, Jovinge S, Fredrikson GN, Vessby B. Conjugated linoleic acid increased C-reactive protein in human subjects. Br J Nutr 2005. 94(5):791-795. [DOD] [CrossRef]
- Sofi F, Buccioni A, Cesari F, Gori AM, Minieri S, Mannini L, Casini A, Gensini GF, Abbate R, Antongiovanni M. Effects of a dairy product (pecorino cheese) naturally rich in cis-9, trans-11 conjugated linoleic acid on lipid, inflammatory and haemorheological variables: a dietary intervention study. Nutr Metab Cardiovasc Dis 2010. 20(2):117-124. [DOD] [CrossRef]
- Contarini G, Povolo M. Phospholipids in milk fat: composition, biological and technological significance, and analytical strategies. Int J Mol Sci 2013. 14(2):2808-2831. [DOD] [CrossRef]
- Eckhardt ER, Wang DQ, Donovan JM, Carey MC. Dietary sphingomyelin suppresses intestinal cholesterol absorption by decreasing thermodynamic activity of cholesterol monomers. Gastroenterology 2002. 122(4):948-956. [DOD] [CrossRef]
- Ohlsson L, Burling H, Duan RD, Nilsson A. Effects of a sphingolipid-enriched dairy formulation on postprandial lipid concentrations. Eur J Clin Nutr 2010. 64(11):1344-1349. [DOD] [CrossRef]
- Keller S, Malarski A, Reuther C, Kertscher R, Kiehntopf M, Jahreis G. Milk phospholipid and plant sterol-dependent modulation of plasma lipids in healthy volunteers. Eur J Nutr 2013. 52(3):1169-1179. [DOD] [CrossRef]
- Conway V, Couture P, Richard C, Gauthier SF, Pouliot Y, Lamarche B. Impact of buttermilk consumption on plasma lipids and surrogate markers of cholesterol homeostasis in men and women. Nutr Metab Cardiovasc Dis 2013. 23(12):1255-1262. [DOD] [CrossRef]
- Conway V, Couture P, Gauthier S, Pouliot Y, Lamarche B. Effect of buttermilk consumption on blood pressure in moderately hypercholesterolemic men and women. Nutrition 2014. 30(1):116-119. [DOD] [CrossRef]
This article has been cited by other articles:
|
Dairy consumption is associated with a lower incidence of the metabolic syndrome in middle-aged and older Korean adults: the Korean Genome and Epidemiology Study (KoGES)
Kim D, Kim J
Br J Nutr 2017. 117(1):148-160
|
|
|
Recent Advances in Phospholipids from Colostrum, Milk and Dairy By-Products
Verardo V, Gomez-Caravaca AM, Arraez-Roman D, Hettinga K
Int J Mol Sci 2017. 18(1):E173
|
|
|
Discrepancy between Hepatitis C Virus Genotypes and NS4-Based Serotypes: Association with Their Subgenomic Sequences
Win NN, Nakamoto S, Kanda T, Takahashi H, Takahashi-Nakaguchi A, Yasui S, Nakamura M, Wu S, Imazeki F, Mikami S, Yokosuka O, Gonoi T, Shirasawa H
Int J Mol Sci 2017. 18(1):E172
|
|
|
Biomarkers of the metabolic syndrome: Influence of minerals, oligo- and trace elements
Robberecht H, Bruyne T, Hermans N
J Trace Elem Med Biol 2016. In press
|
|
|
Is Butter Back? A Systematic Review and Meta-Analysis of Butter Consumption and Risk of Cardiovascular Disease, Diabetes, and Total Mortality
Pimpin L, Wu JH, Haskelberg H, Del Gobbo L, Mozaffarian D
Plos One 2016. 11(6):e0158118
|
|
|
Environmental exposure to BDE47 is associated with increased diabetes prevalence: Evidence from community-based case-control studies and an animal experiment
Zhang Z, Li S, Liu L, Wang L, Xiao X, Sun Z, Wang X, Wang C, Wang M, Li L, Xu Q, Gao W, Wang SL
Sci Rep 2016. 6:27854
|
|
|
The Truth About Dairy Fats
Dennett C
Today Diet 2016. 18(10):26
|
|
|
Co-Ingestion of Whey Protein with a Carbohydrate-Rich Breakfast Does Not Affect Glycemia, Insulinemia or Subjective Appetite Following a Subsequent Meal in Healthy Males
Allerton DM, Campbell MD, Gonzalez JT, Rumbold PL, West DJ, Stevenson EJ
Nutrients 2016. 8(3):116
|
|
|
Macronutrient Composition and Management of Non-Insulin-Dependent Diabetes Mellitus (NIDDM): A New Paradigm for Individualized Nutritional Therapy in Diabetes Patients
Koloverou E, Panagiotakos DB
Rev Diabet Stud 2016. 13(1):6-16
|
|
|
Whey and Casein Proteins and Medium-Chain Saturated Fatty Acids from Milk Do Not Increase Low-Grade Inflammation in Abdominally Obese Adults
Bohl M, Bjørnshave A, Gregersen S, Hermansen K
Rev Diabet Stud 2016. 13(2-3):148-157
|
|
|