Review
Rev Diabet Stud,
2019,
15:1-15 |
DOI 10.1900/RDS.2019.15.1 |
Diabetes and Lung Disease: A Neglected Relationship
Jasmin Khateeb1,2, Eyal Fuchs2,3, Mogher Khamaisi1,4,5
1Department of Internal Medicine D, Rambam Health Care Campus, Haifa, Israel
2Pulmonary Division, Rambam Health Care Campus, Haifa, Israel
3Department of Internal Medicine C, Rambam Health Care Campus, Haifa, Israel
4Faculty of Medicine - Technion - Israel Institute of Technology, Haifa, Israel
5Division of Endocrinology, Diabetes and Metabolism, Haifa, Israel
Address correspondence to: Jasmin Khateeb, e-mail: j_atalla@rambam.health.gov.il
Manuscript submitted August 9, 2018; accepted October 26, 2018.
Keywords: asthma, chronic obstructive pulmonary disease, idiopathic pulmonary fibrosis, pulmonary hypertension, lung cancer, hypoglycemic drugs
Abstract
BACKGROUND: Diabetes mellitus is a systemic disorder associated with inflammation and oxidative stress which may target many organs such as the kidney, retina, and the vascular system. The pathophysiology, mechanisms, and consequences of diabetes on these organs have been studied widely. However, no work has been done on the concept of the lung as a target organ for diabetes and its implications for lung diseases. AIM: In this review, we aimed to investigate the effects of diabetes and hypoglycemic agent on lung diseases, including asthma, chronic obstructive pulmonary disease (COPD), idiopathic pulmonary fibrosis, pulmonary hypertension, and lung cancer. We also reviewed the potential mechanisms by which these effects may affect lung disease patients. RESULTS: Our results suggest that diabetes can affect the severity and clinical course of several lung diseases. CONCLUSIONS: Although the diabetes-lung association is epidemiologically and clinically well-established, especially in asthma, the underlying mechanism and pathophysiology are not been fully understood. Several mechanisms have been suggested, mainly associated with the pro-inflammatory and proliferative properties of diabetes, but also in relation to micro- and macrovascular effects of diabetes on the pulmonary vasculature. Also, hypoglycemic drugs may influence lung diseases in different ways. For example, metformin was considered a potential therapeutic agent in lung diseases, while insulin was shown to exacerbate lung diseases; this suggests that their effects extend beyond their hypoglycemic properties.
Abbreviations: ABCA1 - adenosine triphosphate binding cassette, subfamily A, member 1; ADPN - adiponectin; COPD - chronic obstructive pulmonary disease; CRP - C-reactive protein; DLco - lung diffusing capacity; EMT - epithelial to mesenchymal transition; eNOS - endothelial nitric oxide synthase; FEV1 - forced expiratory volume in 1 second: FVC - forced vital capacity; GERD - gastroesophageal reflux disease; GLP-1 - glucagon-like peptide 1 agonists; GOLD - Global Initiative for Chronic Obstructive Lung Disease; HR - hazard ration; HRCT - high-resolution computed tomography; ILGF-1 - insulin-like growth factor 1; IL-6 - interleukin 6; IPF - idiopathic pulmonary fibrosis; MCP-1 - monocyte chemoattractant protein; MMP-9 - matrix metalloprotinase 9; NADPH - nicotinamide adenine dinucleotide phosphate; NF-κB - nuclear factor kappa-light-chain-enhancer of activated B cells; NO - nitric oxide; NOD - non-obese diabetic; PDGF - platelet-derived growth factor; PH - pulmonary hypertension; PPARγ - peroxisome proliferator-activated receptor gamma; RAGE - receptor for advanced glycation end-products; RV - right ventricular; SGLT2 - sodium-glucose cotransporter 2; SV - stroke volume; TGF-β1 - transforming growth factor beta 1; TNF - tumor necrosis factor; UIP - usual interstitial pneumonia
1. Introduction
Diabetes mellitus is a systemic disorder characterized by a chronic hyperglycemic state that is associated with inflammation and oxidative stress. This leads to micro- and macrovascular damage to many organs, especially the kidney, retina, and cardiovascular system [1-4]. The clinical basis and molecular mechanisms associated with the diabetic micro- and macrovascular damage to these organs have been investigated widely. However, there is a lack of evidence and research regarding the lung as a target for diabetic damage. This is because the clinical significance of such damage is unknown, mainly due to the extensive physiological reserve of the lung.
The lung has a complicated alveolar-capillary network which may be targeted by diabetic microvascular damage, suggesting its involvement in diabetes. Diabetic patients frequently report respiratory symptoms [5] and are at increased risk of several pulmonary diseases [6].
Hyperglycemia has been shown to lead to interstitial fibrosis [7] and alveolar capillary microangiopathy [8]; it is associated with both restrictive and obstructive lung function impairment, including reduction in forced expiratory volume in 1 second (FEV1), forced vital capacity (FVC), lung diffusing capacity (DLco) [9-10], and lung elastic recoil [11]. It has also been shown to cause mucus overproduction in the airway, contributing to morbidity and mortality in many lung diseases [12].
The molecular basis for the diabetes-lung association has yet to be fully investigated and understood. However, several molecular mechanisms have been suggested; they involve mainly pro-inflammatory pathways and vascular inflammation. One such mechanism is the receptor for advanced glycation end-products (RAGE) which is expressed in the lung and promotes vascular inflammation in diabetic patients [13]. Another is interleukin 6, a biomarker of inflammation and metabolic dysfunction, which has been suggested as a severity predictor in lung diseases [14]. Interestingly, little evidence suggests a beneficial role of hypoglycemic agents on lung function and inflammation [15].
In this review, we aim to discuss the effects of diabetes and hypoglycemic agents on lung diseases such as asthma, chronic obstructive pulmonary disease (COPD), idiopathic pulmonary fibrosis (IPF), pulmonary hypertension (PH), and lung cancer, and we review the potential mechanisms by which they may affect lung disease patients.
2.1 Background
2. Asthma and diabetes
Asthma is a heterogeneous disease mainly characterized by airway hyperresponsiveness, which leads to bronchoconstriction and chronic inflammation. This causes proliferation and extracellular matrix deposition, eventually leading to the destruction of the airway wall structure and airway remodeling. Asthma manifests mainly by two phenotypes, distinguished by their inflammatory responses [16]:
- Th2-predominant inflammation, typically atopic, which leads to early-onset asthma that responds well to steroids.
- Adult-onset asthma that typically occurs in non-atopic patients with Th1-predominance, which is more closely related to metabolic and inflammatory processes such as diabetes [17].
Table 1 provides an overview of lung diseases in diabetic patients.
Table
1.
Lung diseases in diabetic patients |
|
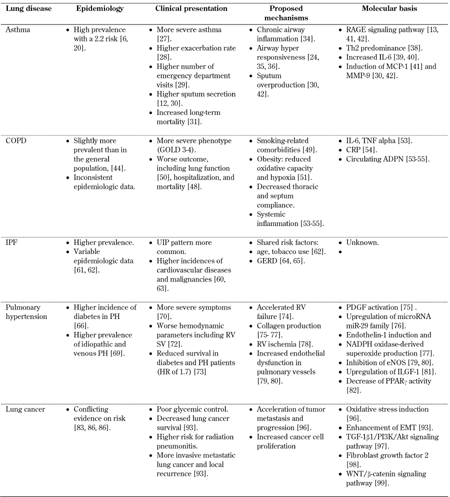 |
 |
Legend:
ADPN - adiponectin, COPD - chronic obstructive pulmonary disease, CRP – C-reactive protein, EMT - epithelial to mesenchymal transition, eNOS - endothelial nitric oxide synthase, GERD - gastro-esophageal reflux disease, IL-6 - interleukin 6, ILGF-1 - insulin-like growth factor, IPF - idiopathic pulmonary fibrosis, MCP-1 - monocyte chemoattractant protein, MMP-9 - matrix metalloprotinase 9, PDGF – platelet-derived growth factor, PH - pulmonary hypertension, PPARγ - peroxisome proliferator-activated receptor gamma, RAGE - receptor for advanced glycation end-products, RV - right ventricular, SV - stroke volume, TNF - tumor necrosis factor, UIP - usual interstitial pneumonia. |
|
2.2 Epidemiology
Earlier studies reported an inverse relationship between atopy and diabetes mellitus [18]. Type 1 diabetes was associated with a lower incidence of asthma, which was due to the anti-inflammatory properties of insulin [19]. In contrast, later studies found high levels of asthma in countries with a high level of type 1 diabetes [20].
The association between type 2 diabetes and asthma has been investigated in more detail. Type 2 diabetes has been shown to cause airway hyperresponsiveness in humans [21], with an association between insulin resistance and increased risk of asthma-like symptoms [22]. The risk of asthma in diabetic patients is over twice that of non-diabetics (hazard ratio (HR) of 2.2) [6]. A higher prevalence of asthma has been found in hospitalized patients with type 2 diabetes, independent of other comorbid conditions [23]. In one study, it was shown that asthma symptoms preceded the diagnosis of diabetes by a few years, regardless of glucocorticoid treatment [24]. Likewise, uncontrolled diabetes has been associated with a higher risk of asthma, and poor glycemic control has been related to increased asthma risk [25]. In contrast, a large prospective study found no significant statistical relationship among women with asthma [26], suggesting that the diabetes-asthma association is still not sufficiently well established.
2.3 Clinical presentation
Diabetes in asthma patients is an important comorbidity, causing more severe asthma [27] with a higher exacerbations rate [28] and more frequent emergency department visits [29]. Diabetes was also shown to induce sputum hypersecretion [12, 30]. Moreover, diabetes in the context of asthma exacerbation was shown to impact long-term mortality [31].
2.4 Mechanisms
Chronic inflammation and pro-inflammatory cytokines may emerge in the pathogenesis of both diabetes mellitus [32] and asthma [33]. It has been shown that diabetes is a pro-inflammatory state associated with airway inflammation [34]. Studies on type 2 diabetes have shown that it causes airway hyperresponsiveness in human airway smooth muscle cells in vitro [35], in diabetic animal models [36], and in humans [25]. Therefore, the most investigated pathway in the pathogenesis of the diabetes-asthma association is chronic inflammation such as RAGE.
RAGE signaling has been shown to be highly expressed in the lung and to induce chronic airway and vascular inflammation [13, 37]. RAGE has a regulatory role in T-cell proliferation and differentiation of both Th1 and Th2 cells [38]. Diabetes-prone non-obese diabetic (NOD) mice have been shown to give rise to enhanced Th2-mediated responses and contribute to a Th2-predominant asthma phenotype. Increased systemic interleukin 6 as an inflammatory and metabolic dysfunction biomarker in diabetes has been associated with more severe asthma [39, 40]. In this context, monocyte chemoattratant protein (MCP) 1, which recruits monocytes to inflammation sites, has been shown to play a significant role in diabetic patients with asthma via airway remodeling and predicts a poorer prognosis [41].
Matrix metallopreinase (MMP) 9 mediates sputum overproduction secondary to airway epithelial barrier dysfunction caused by hyperglycemia, especially during exacerbation, and cause airway inflammation in airway epithelial cells [30, 42].
2.5 Summary
The diabetes-asthma association is well-established epidemiologically and clinically. Diabetes is a risk factor for more severe and complicated asthma. The main pathogenesis of this association is inflammation and pro-inflammatory cytokines. However, the pathogenesis has yet to be fully understood and more research is necessary to establish a strong biological basis.
3. Chronic obstructive pulmonary disease (COPD) and diabetes
3.1 Introduction
COPD is a preventable and treatable disease characterized by persistent respiratory symptoms and airflow limitation due to airway and/or alveolar abnormalities, which are often secondary to significant exposure to noxious particles or gases [43]. It is currently the fourth leading cause of death worldwide.
It has been increasingly recognized that the presence of common factors in COPD and in other chronic extra-pulmonary diseases, such as diabetes mellitus, together with the frequent coexistence of these conditions in the same adult individual, supports the hypothesis of common processes sharing their pathogeneses within the same patient [44].
3.2 Epidemiology
Metabolic syndrome has been recognized as one of the most relevant clinical comorbidities associated with COPD patients [45]. However, the link between COPD and diabetes is much less clear. Diabetes is more prevalent in COPD than in the general population. Prevalence estimates of diabetes among COPD patients range between 10.1-23.0% [46, 47].
3.3 Clinical presentation
The risk of diabetes in COPD patients has been found to be higher in more severe phenotypes (level 3-4 according to the Global Initiative for Chronic Obstructive Lung Disease (GOLD) guideline). This risk was independent of BMI, smoking, and other confounding factors. Moreover, the presence of diabetes among those with COPD has been shown to be associated with worse outcomes, such as mortality and hospitalization [48].
3.4 Mechanisms
The mechanisms by which diabetes influences lung function have not yet been fully determined. The correlation between COPD and diabetes may depend on several mutual risk factors and physiological alterations.
COPD patients are primarily former or active smokers. Smoking may lead to concomitant comorbidity, but it is increasingly evident that patients with COPD also have a high burden of comorbidity independent of smoking [49]. In the large COPD Gene cohort, diabetes subjects with a history of smoking had worse lung function even if they had no established diagnosis of COPD [50].
Recently, there has been increasing interest in the relationship between obesity and COPD, although the nature of this association remains unknown. It has been proposed that reduced oxidative capacity and systemic hypoxia may play a role in the pathogenesis of COPD in obese patients [51]. The potential interaction between impaired adipose tissue function, systemic inflammation, and COPD may provide insight into the pathogenesis and reversibility of the systemic pathology in the disease. The effects of obesity on respiratory function depend on the mass and anatomical distribution of the excessive adipose tissue in the thorax and abdomen [52]. Thus, another potential explanation is that increased abdominal obesity directly affects thoracic and diaphragm (septum?) compliance, which impairs lung function.
COPD is associated with a chronic systemic inflammatory condition. Insulin resistance has been found to be related to interleukin 6 (IL-6) and tumor necrosis factor alpha soluble receptor in non-hypoxemic COPD patients [53]. Moreover, elevated serum levels of C-reactive protein (CRP) have also been associated with impaired pulmonary function [54]. Increased visceral fat has been identified as the main factor increasing CRP concentration [55]. Another mechanism associated with COPD is circulating adiponectin (ADPN), which has been inversely associated with disease severity in patients with COPD. Studies assessing the relationship between ADPN and lung function in subjects from the general population have shown diverging results. It is noteworthy that there was no association between ADPN and lung function after adjustment for covariates related to adiposity [56].
3.5 Summary
The association between COPD and diabetes has not been fully established epidemiologically and clinically. However, it is strongly suggested that continuously elevated levels of inflammatory mediators, reflecting the enhanced inflammatory state seen in COPD, may contribute to the development of diabetes. Whilst a converse relation is not suggested; the presence of diabetes alone is not considered a risk factor for COPD, and has not been found to contribute to its development in studies regarding COPD pathogenesis.
4. Idiopathic pulmonary fibrosis (IPF) and diabetes
4.1 Introduction
IPF is defined as a specific form of chronic, progressive, fibrosing interstitial pneumonia of unknown cause, occurring primarily in older adults. It is characterized by progressive worsening of dyspnea and lung function and is associated with a poor prognosis [57]. No significant extra-pulmonary manifestation has been recognized so far. Thus, it is assumed to be limited to the lung.
4.2 Epidemiology
The incidence of IPF is estimated to be between 4 to 11 cases per 100,000 persons per year, and is more common in males. It increases with older age, typically occurring in the sixth and seventh decade. The majority of patients have a history of cigarette smoking [58, 59]. Although considered a single-organ disease (affecting only the lung), many studies suggest that there is an association between IPF and diabetes [60, 61]. In a nationwide Korean survey performed in the years 2003-2007, which retrospectively analyzed 1,685 patients using the interstitial lung disease (ILD) registry, 17.8% of the patients with IPF also had diabetes [60].
Case-control analyses performed in Japan, Mexico, and the U.K. estimated the prevalence of type 2 diabetes among individuals with IPF to be 10-33%, which was significantly higher than that of matched control populations [62].
4.3 Clinical presentation
Many comorbid conditions are known to occur in IPF, including coronary artery disease, pulmonary hypertension, gastroesophageal reflux, and diabetes [63]. It remains unclear whether the presence of diabetes influences survival in patients with IPF. However, IPF patients with diabetes are more likely to have the usual interstitial pneumonia (UIP) patterns on high-resolution computed tomography (HRCT), including reticular and honeycomb patterns, than are those without diabetes. Furthermore, significantly higher incidences of hypertension, cardiovascular diseases, and other malignancies (except lung cancer) have been found in IPF patients with diabetes than in IPF patients without diabetes [60].
4.4 Mechanisms
Since the incidence of IPF increases with age, it is possible that age and lifestyle-related diseases, including diabetes, may be a risk factor affecting either the initiation or progression of IPF. In a case-controlled study, the adjusted odds ratios for cigarette smoking and diabetes were 5.40 (95% CI: 2.30-12.66) and 4.06 (95% CI: 1.80-9.15) [62]. Another potential link between IPF and diabetes is based on the higher prevalence of gastroesophageal reflux disease (GERD) in both conditions. Patients with diabetes are at greater risk of GERD than those without diabetes. [64]. Several studies suggest that GERD is a risk factor for IPF because of its presumed association with microaspiration. Abnormal GERD is common in patients with IPF [65].
4.5 Summary
It has yet to be defined whether diabetes is associated with IPF. However, mounting evidence suggests at least an epidemiologic connection with these conditions and a trend towards a more severe disease state.
5. Pulmonary hypertension (PH) and diabetes
5.1 Introduction
PH is an abnormally elevated pressure in the pulmonary vasculature that may cause right heart failure and death. The micro- and macrovascular damage caused by diabetes may affect the pulmonary vasculature, suggesting that diabetes may play a role in the development and exacerbation of pulmonary hypertension. It has been shown that the incidence of diabetes in PH patients is higher than in the general population, suggesting a connection between the two diseases [66]. However, the clinical significance is unknown, mostly because of the extensive reserve within the pulmonary capillary bed.
5.2 Epidemiology
It has not yet been established whether pulmonary hypertension is the consequence of diabetes or its cause. It has been shown that PH patients have more glucose intolerance [67], and that diabetic patients were more likely to have idiopathic pulmonary hypertension [68] (24% compared to 10%), and to have venous rather than arterial pulmonary hypertension [69].
5.3 Clinical presentation
Symptoms of PH patients and physical performance measured by a 6-minute walk test were worse in diabetic than in non-diabetic patients [70]. In patients with COPD and diabetes, PH was more severe than in patients with COPD only [71]. Moreover, hemodynamic parameters such as right atrial pressure and mean pulmonary capillary wedge pressure were higher in diabetic patients than in non-diabetics, with a trend towards lower response to nitric oxide (NO) in diabetic patients [69]. It was shown that right ventricular stroke volume, which is associated with PH prognosis and survival, was reduced in patients with diabetes and PH [72]. Consequently, survival among PH patients with diabetes was lower than that of patients without diabetes (hazard ratio of 1.7) [73]. It was also obvious that well-controlled diabetes (HbA1c less than 5.7) in PH patients was associated with greater survival [74].
5.4 Mechanisms
Although mechanisms that contribute to the development of pulmonary hypertension have been widely studied, the effect of diabetes on pulmonary vasculature is less well understood. It has been shown that diabetes influences PH by causing accelerated right heart failure [74]. Diabetes has been shown to accelerate fibrosis of the right heart via platelet-derived growth factor (PDGF) activation, which causes an increase in the transforming growth factor beta 1 (TGF-β1) gene, thus modulating both human mesangial cells and matrix [75]. Another mechanism is via upregulation of the microRNA miR-29 family, which causes collagen production by cardiac fibroblasts [76]. Moreover, hyperglycemia has been shown to induce endothelin-1 in the right ventricle, and to cause fibrosis [77].
It has also been suggested that right ventricle ischemia plays a role in PH patients with diabetes, which is mostly due to the role of diabetes in the general promotion of ischemia [78]. However, there have been no specific studies on the role of diabetes in ischemia of pulmonary vasculature. Animal studies suggest that diabetes may have a direct effect on pulmonary vasculature. It has been found that pulmonary arteries from diabetic rats are less responsive to vasodilatation, because of increased endothelial dysfunction via enhanced nicotinamide adenine dinucleotide phosphate (NADPH) oxidase-derived superoxide production and inhibition of endothelial nitric oxide synthase (eNOS) [79, 80].
Diabetes has also been shown to increase arterial smooth muscle cell proliferation via upregulation of insulin-like growth factor 1 (ILGF-1) [81]. Also, the anti-proliferative role of peroxisome proliferator-activated receptor gamma (PPARγ) was decreased in diabetes, while its effect was enhanced with the PPARγ activator rosiglitazone [82].
5.5 Summary
Diabetes and pulmonary hypertension are strongly associated. Diabetic microvascular and macrovascular injuries may affect pulmonary vasculature by increasing its susceptibility to the development and progression of pulmonary hypertension, and may play a role in patient prognosis and survival.
6. Lung cancer and diabetes
6.1 Introduction
Recent studies suggest an association between diabetes and lung cancer, providing epidemiological and clinical support for the hypothesis that diabetes is a risk factor for lung cancer [90]. Diabetes may influence lung cancer progression and outcome, and may serve as a poor prognostic factor for lung cancer [83].
6.2 Epidemiology
Type 1 diabetes is regarded to be associated with an increased risk of lung cancer [84], despite several conflicting retrospective studies on lung cancer prognosis among diabetic patients [85, 86]. On the basis of two large cohorts from Shanghai, it was reported that the risk of lung cancer was observed in both men and women, with a HR of 0.87 and 0.92, respectively [87]. However, a recent large prospective study provided evidence that pre-existing diabetes is associated with poor survival among women with cancer [88], and it is considered a negative prognostic factor in lung cancer [89].
6.3 Clinical presentation
Proper glycemic control for lung cancer patients is required to induce antineoplastic effects and increase survival (HZ of 0.62) [90]. Diabetic patients have been shown to be more vulnerable to radiation [91]. Diabetes has also been shown to be a risk factor for radiation pneumonitis in lung cancer patients who receive radiotherapy [92]. A multi-center study found that diabetic patients with lung cancer may have a more invasive metastatic lung cancer with greater local recurrence [93].
On the contrary, several studies found that diabetes does not impact overall survival in lung cancer [94], and may even be associated with longer survival rates [85]. Furthermore, another study found that diabetes may play a protective role against lung metastasis [95], suggesting that the role of diabetes in lung cancer needs further clarification.
6.4 Mechanisms
Diabetes may influence cancer progression and prognosis via several mechanisms. It has been shown to accelerate tumor metastasis and tumor progression in a lung cancer animal model via hyperglycemia-induced oxidative stress. This effect is reversible when removing systemic hydrogen peroxide [96]. Diabetes has been shown to increase cancer invasiveness via enhancement of epithelial to mesenchymal transition (EMT), which plays a key role in local tumor recurrence and metastasis in non-small cell lung cancer [93], and via an increased metastasis-associated protein expression secondary to oxidative stress and increased upregulation of TGF-1β1/PI3K/Akt signaling pathway [97].
Consequently, it has been shown that hyperglycemia enhances cell proliferation via fibroblast growth factor 2, and that hyperglycemia may alter endothelial cell function and promote basement membrane changes. It may also promote cancer cell proliferation [98] via metabolic remodeling, resulting in WNT/β-catenin signaling pathway enhancement, thus promoting proliferation, survival, and senescence bypass [99].
6.5 Summary
Epidemiological and clinical data support an association between diabetes and lung cancer. The pre-existence of diabetes in lung cancer is assumed to aggravate lung cancer, although this remains unclear.
7. Lung diseases and hypoglycemic agents
7.1 Introduction
The diabetes-lung association hypothesis has been studied in combination with the effect of hypoglycemic drugs on the lungs (Table 2). Interestingly, hypoglycemic drugs seem to have a role beyond their contribution to diabetes control; they also act as a modulator of airway glucose homeostasis, leading to lung disease exacerbation or prevention [100].
Table
2.
Hypoglycemic agents and lung diseases |
|
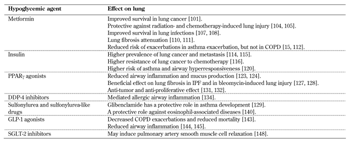 |
 |
Legend:
COPD - chronic obstructive pulmonary disease, DPP-4 - dipeptidyl peptidase 4, GLP-1 - glucagon-like peptide 1, IPF - idiopathic pulmonary fibrosis, PPARγ - peroxisome proliferator-activated receptor gamma, SGLT2 - sodium-glucose cotransporter 2. |
|
7.2 Metformin
The most common oral antidiabetic drug and most investigated in lung diseases is metformin, mainly because of its antidiabetic, antioxidant, and anti-inflammatory properties.
The role of metformin in lung cancer is constantly under debate. In a meta-analysis of the association between hypoglycemic agents and lung cancer prognosis, metformin was the only drug shown to improve survival outcomes [101], especially in non-small cell lung cancer [102]. It has also been shown to have anticancer effects beyond its role as a drug for diabetes [103], such as a protective agent for both radiation-induced pulmonary injury [104] and chemotherapy pneumonitis [105]. In contrast, a recent large cohort study concluded that, unlike breast and liver cancer, there is no evidence of an antitumor efficacy of metformin on lung cancer [106]. Thus, the protective role of metformin on lung cancer is still unknown.
In lung infections, metformin has been shown to promote macrophage bactericidal activity and improve survival [107, 108]; and it may serve both as a means of protection against pulmonary tuberculosis and as a therapy for improving the effectiveness of anti-tuberculous drugs [109]. In idiopathic pulmonary fibrosis, metformin attenuated lung fibrosis via inhibition of fibrosis marker expression, and it has been proposed as an anti-fibrotic modality [110, 111]. Metformin use in asthmatic patients has been associated with a significant reduction in asthma exacerbations and hospitalization [112]. In COPD, metformin seems to have a protective role on lung inflammatory response during the development of emphysema [113]. However, it had no effect on COPD exacerbations [15].
To conclude, metformin has a beneficial role in various lung diseases independent of its antidiabetic role, and it may be considered as a potential therapeutic agent for clinical use in lung disease. However, its role in lung cancer prevention remains unknown.
7.3 Insulin
In contrast to metformin, insulin is known to have oncogenic activity, and it is associated with non-small cell lung cancer development [114]. Insulin-like growth factor receptor 1 (IGF-1) is associated with lung cancer development and metastasis [115] and an acquired resistance to chemotherapy [116].
Insulin has been shown to modify mast cell phenotype and increase its activity in vitro [117], along with increasing hyperresponsiveness, bronchoconstriction [118, 119], and airway inflammation [19]. Insulin modulates T-cell differentiation by promoting a shift towards Th2 type response, which is the main disease pathway in asthma [120]. A recent study in a Taiwanese cohort demonstrated that insulin may increase the risk of asthma [121]. Although very little is known regarding the association between insulin and IPF, it has been suggested that insulin growth factor binding proteins may be a key factor responsible for IPF initiation [122].
In conclusion, we assume that insulin affects the lung by causing airway inflammation, exacerbating lung disease, and playing a role in lung cancer development.
7.4 PPAR-γ agonists
Several studies suggest that PPAR-γ agonists reduce airway inflammation [123] and decrease mucus secretion [124]. It has been shown that pioglitazone diminishes alveolar and interstitial neutrophil influx and reduces lung inflammation and injury both in vitro [125] and in animal models and that it attenuates lung ischemia reperfusion injury via inhibition of pro-inflammatory cytokines [126]. Pioglitazone has been shown to have a beneficial effect on fibrotic processes of the lung, suggesting that it may serve as an anti-fibrotic agent in IPF and in bleomycin-induced lung injury [127, 128].
Rosiglitazone, another PPAR-γ agonist, demonstrated beneficial effects on lung function and airway inflammation in a rat model of asthma [129]. However, this effect was not achieved in human studies in vivo [130].
Regarding lung cancer, PPAR-γ agonists have demonstrated anti-tumor and anti-proliferative properties [131, 132] and might offer therapeutic effects in lung cancer [133].
7.5 DPP-4 inhibitors
These drugs were shown in vitro and in an animal model to mediate allergic airway inflammation and regulate common immunological pathways in asthma via CD26 [134]. However, no association between DPP-4 use and asthma control was found [135].
7.6 Sulfonylurea and sulfonylurea-like drugs
Although a large-scale study showed that insulin secretagogues increased the risk of overall cancer [136], a recent meta-analysis showed that sulfonylurea use was not associated with risk of lung cancer [137]. In another study, sulfonylurea use was proposed in combination with chemotherapy for resistant lung cancer cells [138]. Glibenclamide has been shown to play an important protective role in asthma development via airway muscle relaxation in mice [139], and glyburide inhibited cytokine-mediated eosinophil survival and superoxide production, suggesting that it could be used to treat eosinophil-associated disease, such as asthma [140].
7.7 Glucagon-like peptide 1 agonists (GLP-1)
The GLP-1 receptor is found in human lung tissue [141], suggesting that the lung may be a target for GLP-1 agonists. GLP-1 augments surfactant production in rats [142] and was shown to be a potential therapy in the treatment of obstructive pulmonary disease by decreasing severity of acute exacerbations and reducing mortality in an animal model of obstructive lung disease [143]. The mechanism is still unknown, but it has been suggested that GLP-1 may have anti-inflammatory effects via downregulation of inflammatory cytokines, such as TNFα and NF-κB [144, 145], and via airway smooth muscle cell proliferation and migration mediated by adenosine triphosphate binding cassette, subfamily A, member 1 (ABCA1) [146]. However, the effect of GLP-1 agonists on human lungs has not yet been investigated, and clinical data are lacking.
7.8 Inhibitors of sodium-glucose cotransporter 2 (SGLT2)
These are a new class of oral anti-diabetic drugs with increasing evidence of a beneficial role in cardiovascular diseases [147]. Little is known about their effects on the lung. A recent in vitro study suggested that SGLT2 may induce human pulmonary artery smooth muscle cell relaxation in an NO-dependent manner [148]. However, their role in pulmonary vasculature and lung diseases has yet to be investigated.
8. Conclusion
Diabetes mellitus is one of the most thoroughly investigated systemic diseases worldwide, and is considered a part of the metabolic syndrome epidemic. Although it has been shown to affect almost every organ in the body, the lung is one of the most neglected target organs of diabetes, presumably because its clinical relevance is undetermined. Thus, the diabetes-lung association is considered a newly investigated concept. In fact, most pulmonology literature does not address diabetes as an influencing factor for lung diseases.
Emerging evidence suggests that diabetes and the widely used hypoglycemic drugs may affect the pathogenesis, development, and progression of several lung diseases and their prognosis and clinical outcome, suggesting that diabetes should be considered as a relevant factor in the clinical approach to lung disease patients. The pro-inflammatory, proliferative, and oxidative properties of hyperglycemia have been shown to have an important role in affecting pulmonary vasculature, airways, and lung parenchyma.
The evidence reviewed in this article supports further investigation in this field. More studies are needed to evaluate and reinforce the biological and clinical associations between diabetes and lung disease, and thus more research is justified. After all, why should the lung be a neglected organ within this systemic and epidemic disease?
Disclosures: The authors report no conflict of interests.
References
- Folli F, Corradi D, Fanti P, Davalli A, Paez A, Giaccari A, Perego C, Muscogiuri G. The role of oxidative stress in the pathogenesis of type 2 diabetes mellitus micro- and macrovascular complications: avenues for a mechanistic-based therapeutic approach. Curr Diabetes Rev 2011. 7(5):313-324. [DOD] [CrossRef]
- Kashihara N, Haruna Y, Kondeti VK, Kanwar YS. Oxidative stress in diabetic nephropathy. Curr Med Chem 2010. 17(34):4256-4269. [DOD] [CrossRef]
- Fujita T, Hemmi S, Kajiwara M, Yabuki M, Fuke Y, Satomura A, Soma M. Complement-mediated chronic inflammation is associated with diabetic microvascular complication. Diabetes Metab Res Rev 2013. 29(3):220-226. [DOD] [CrossRef]
- Kowluru RA, Chan PS. Oxidative stress and diabetic retinopathy. Exp Diabetes Res 2007. 2007:43603. [DOD]
- De Santi F, Zoppini G, Locatelli F, Finocchio E, Cappa V, Dauriz M, Verlato G. Type 2 diabetes is associated with an increased prevalence of respiratory symptoms as compared to the general population. BMC Pulm Med 2017. 17(1):101. [DOD] [CrossRef]
- Ehrlich SF, Quesenberry CP Jr, Van Den Eeden SK, Shan J, Ferrara A. Patients diagnosed with diabetes are at increased risk for asthma, chronic obstructive pulmonary disease, pulmonary fibrosis, and pneumonia but not lung cancer. Diabetes Care 2010. 33(1):55-60. [DOD] [CrossRef]
- Zou XZ, Gong ZC, Liu T, He F, Zhu TT, Li D, Zhang WF, Jiang JL, Hu CP. Involvement of epithelial-mesenchymal transition afforded by activation of LOX-1/TGF-beta1/KLF6 signaling pathway in diabetic pulmonary fibrosis. Pulm Pharmacol Ther 2017. 44:70-77. [DOD] [CrossRef]
- Williams JG, Morris AI, Hayter RC, Ogilvie CM. Respiratory responses of diabetics to hypoxia, hypercapnia, and exercise. Thorax 1984. 39(7):529-534. [DOD] [CrossRef]
- Fontaine-Delaruelle C, Viart-Ferber C, Luyton C, Couraud S. Lung function in patients with diabetes mellitus. Rev Pneumol Clin 2016. 72(1):10-16. [DOD] [CrossRef]
- Nakajima K, Kubouchi Y, Muneyuki T, Ebata M, Eguchi S, Munakata H. A possible association between suspected restrictive pattern as assessed by ordinary pulmonary function test and the metabolic syndrome. Chest 2008. 134(4):712-718. [DOD] [CrossRef]
- Hamlin CR, Kohn RR, Luschin JH. Apparent accelerated aging of human collagen in diabetes mellitus. Diabetes 1975. 24:902-904. [DOD] [CrossRef]
- Vestbo J. Epidemiological studies in mucus hypersecretion. Novartis Found Symp 2002. 248:3-12. [DOD]
- Brett AM, Schmidt SD, Yan YS, Zou E, Weidman D, Pinsky D, Nowygrod R, Neeper M, Przysiecki C, Shaw A, et al. Survey of the distribution of a newly characterized receptor for advanced glycation end products in tissues. Am J Pathol 1993. 143(6):1699-1712. [DOD]
- Ferreira MA, Matheson MC, Duffy DL, Marks GB, Hui J, Le Souëf P, Danoy P, Baltic S, Nyholt DR, Jenkins M, et al. Identification of IL6R and chromosome 11q13.5 as risk loci for asthma. Australian Asthma Genetics Consortium. Lancet 2011. 378(9795):1006-1014. [DOD] [CrossRef]
- Hitchings AW, Lai D, Jones PW, Baker EH. Metformin in COPD Trial Team. Metformin in severe exacerbations of chronic obstructive pulmonary disease: a randomised controlled trial. Thorax 2016. 71(7):587-593. [DOD] [CrossRef]
- Jones TL, Neville DM, Chauhan AJ. Diagnosis and treatment of severe asthma: a phenotype-based approach. Clin Med (Lond) 2018. 18(Suppl 2):s36-s40. [DOD] [CrossRef]
- Hirano T, Matsunaga K. Late-onset asthma: current perspectives. J Asthma Allergy 2018. 11:19-27. [DOD]
- Swern N. Incidence of diabetes mellitus in asthmatic patients. J Allergy 1931. 2(5):375-378. [DOD] [CrossRef]
- Belmonte KE, Fryer AD, Costello RW. Role of insulin in antigen-induced airway eosinophilia and neuronal M2 muscarinic receptor dysfunction. J Appl Physiol (1985) 1998. 85(5):1708-1718. [DOD] [CrossRef]
- Stene LC, Nafstad P. Relation between occurrence of type 1 diabetes and asthma. Lancet 2001. 357:607-608. [DOD] [CrossRef]
- Dandona P, Ghanim H, Monte SV, Caruana JA, Green K, Abuaysheh S, Lohano T, Schentag J, Dhindsa S, Chaudhuri A. Increase in the mediators of asthma in obesity and obesity with type 2 diabetes: reduction with weight loss. Obesity (Silver Spring) 2014. 22(2):356-362. [DOD] [CrossRef]
- Thuesen BH, Husemoen LL, Hersoug LG, Pisinger C, Linneberg A. Insulin resistance as a predictor of incident asthma-like symptoms in adults. Clin Exp Allergy 2009. 39(5):700-707. [DOD] [CrossRef]
- Glück J, Rogala B. Coexistence of bronchial asthma and diabetes mellitus type 2--retrospective analysis. Pol Arch Med Wewn 1999. 101(1):39-43. [DOD]
- Thomsen SF, Duffy DL, Kyvik KO, Skytthe A, Backer V. Risk of asthma in adult twins with type 2 diabetes and increased body mass index. Allergy 2011. 66(4):562-568. [DOD] [CrossRef]
- Hsiao YT, Cheng WC, Liao WC, Lin CL, Shen TC, Chen WC, Chen CH, Kao CH. Type 1 diabetes and increased risk of subsequent asthma: a nationwide population-based cohort study. Medicine (Baltimore) 2015. 94(36):e1466. [DOD] [CrossRef]
- Rana JS, Mittleman MA, Sheikh J, Hu FB, Manson JE, Colditz GA, Speizer FE, Barr RG, Camargo CA Jr. Chronic obstructive pulmonary disease, asthma, and risk of type 2 diabetes in women. Diabetes Care 2004. 27(10):2478-2484. [DOD] [CrossRef]
- Yeryomenko GV. The dependence of the endothelial function on comorbid states in patients with asthma. Wiad Lek 2018. 71(2 pt 1):329-332. [DOD]
- Price D, Wilson AM, Chisholm A, Rigazio A, Burden A, Thomas M, King C. Predicting frequent asthma exacerbations using blood eosinophil count and other patient data routinely available in clinical practice. J Asthma Allergy 2016. 9:1-12. [DOD] [CrossRef]
- Miller MK, Lee JH, Blanc PD, Pasta DJ, Gujrathi S, Barron H, Wenzel SE, Weiss ST, TENOR Study Group. TENOR risk score predicts healthcare in adults with severe or difficult-to-treat asthma. Eur Respir J 2006. 28(6):1145-1155. [DOD] [CrossRef]
- Yu H, Yang J, Xiao Q, Lü Y, Zhou X, Xia L, Nie D. Regulation of high glucose-mediated mucin expression by matrix metalloproteinase-9 in human airway epithelial cells. Exp Cell Res 2015. 333(1):127-135. [DOD] [CrossRef]
- Koskela HO, Salonen PH, Romppanen J, Niskanen L. A history of diabetes but not hyperglycaemia during exacerbation of obstructive lung disease has impact on long-term mortality: a prospective, observational cohort study. BMJ Open 2015. 5(1):e006794. [DOD] [CrossRef]
- Festa A, D'Agostino R Jr, Tracy RP, Haffner SM. Insulin Resistance Atherosclerosis Study. Elevated levels of acute-phase proteins and plasminogen activator inhibitor-1 predict the development of type 2 diabetes: the insulin resistance atherosclerosis study. Diabetes 2002. 51(4):1131-1137. [DOD] [CrossRef]
- Renauld JC. New insights into the role of cytokines in asthma. J Clin Pathol 2001. 54(8):577-589. [DOD] [CrossRef]
- Mulrennan S, Baltic S, Aggarwal S, Wood J, Miranda A, Frost F, Kaye J, Thompson PJ. The role of receptor for advanced glycation end products in airway inflammation in CF and CF related diabetes. Sci Rep 2015. 5:8931. [DOD] [CrossRef]
- Cazzola M, Calzetta L, Rogliani P, Lauro D, Novelli L, Page CP, Kanabar V, Matera MG. High glucose enhances responsiveness of human airways smooth muscle via the Rho/ROCK pathway. Am J Respir Cell Mol Biol 2012. 47(4):509-516. [DOD] [CrossRef]
- Araujo LM, Lefort J, Nahori MA, Diem S, Zhu R, Dy M, Leite-de-Moraes MC, Bach JF, Vargaftig BB, Herbelin A. Exacerbated Th2 mediated airway inflammation and hyperresponsiveness in autoimmune diabetes-prone NOD mice: a critical role for CD1d-dependent NKT cells. Eur J Immunol 2004. 34(2):327-335. [DOD] [CrossRef]
- Watanabe T, Asai K, Fujimoto H, Tanaka H, Kanazawa H, Hirata K. Increased levels of HMGB-1 and endogenous secretory RAGE in induced sputum from asthmatic patients. Respir Med 2011. 105(4):519-525. [DOD] [CrossRef]
- Akirav EM, Henegariu O, Preston-Hurlburt P, Schmidt AM, Clynes R, Herold KC. The receptor for advanced glycation end products (RAGE) affects T cell differentiation in OVA induced asthma. Plos One 2014. 9(4):e95678. [DOD] [CrossRef]
- Peters MC, McGrath KW, Hawkins GA, Hastie AT, Levy BD, Israel E, Phillips BR, Mauger DT, Comhair SA, Erzurum SC, et al. Plasma interleukin-6 concentrations, metabolic dysfunction, and asthma severity: a cross-sectional analysis of two cohorts. Lancet Respir Med 2016. 4(7):574-584. [DOD] [CrossRef]
- Yokoyama A, Kohno N, Fujino S, Hamada H, Inoue Y, Fujioka S, Ishida S, Hiwada K. Circulating interleukin-6 levels in patients with bronchial asthma. Am J Respir Crit Care Med 1995. 151(5):1354-1358. [DOD] [CrossRef]
- Sindhu S, Koshy M, Al-Roub AA, Akhter N, Al Zanki S, Ali S, Devarajan S, Ahmad R. Differential association of plasma monocyte chemoattractant protein-1 withsystemic inflammatory and airway remodeling biomarkers in type-2 diabetic patients with and without asthma. J Diabetes Metab Disord 2016. 15:40. [DOD] [CrossRef]
- Lemjabbar H, Gosset P, Lamblin C, Tillie I, Hartmann D, Wallaert B, Tonnel AB, Lafuma C. Contribution of 92 kDa gelatinase/type IV collagenase in bronchial inflammation during status asthmaticus. Am J Respir Crit Care Med 1999. 159(4 Pt 1):1298-1307. [DOD] [CrossRef]
- Global Initiative for Chronic Obstructive Lung Disease. Global Strategy for Diagnosis, Management, and Prevention of COPD, 2018. [DOD]
- Fabbri LM, Luppi F, Beghe B, Rabe KF. Complex chronic comorbidities of COPD. Eur Respir J 2008. 31(1):204-212. [DOD] [CrossRef]
- Breyer MK, Spruit MA, Hanson CK, Franssen FM, Vanfleteren LE, Groenen MT, Bruijnzeel PL, Wouters EF, Rutten EP. Prevalence of metabolic syndrome in COPD patients and its consequences. Plos One 2014. 9(6):e98013. [DOD] [CrossRef]
- Parappil A, Depczynski B, Collett P, Marks GB. Effect of comorbid diabetes on length of stay and risk of death in patients admitted with acute exacerbations of COPD. Respirology 2010. 15(6):918-922. [DOD] [CrossRef]
- Rogliani P, Calzetta L, Segreti A, Barrile A, Cazzola M. Diabetes mellitus among outpatients with COPD attending a university hospital. Acta Diabetol 2014. 51(6):933-940. [DOD] [CrossRef]
- Mannino DM, Thorn D, Swensen A, Holguin F. Prevalence and outcomes of diabetes, hypertension and cardiovascular disease in COPD. Eur Respir J 2008. 32(4):962-969. [DOD] [CrossRef]
- Putcha N, Drummond MB, Wise RA, Hansel NN. Comorbidities and chronic obstructive pulmonary disease: prevalence, influence on outcomes, and management. Semin Respir Crit Care Med 2015. 36(4):575-591. [DOD] [CrossRef]
- Kinney GL, Black-Shinn JL, Wan ES, Make B, Regan E, Lutz S, Soler X, Silverman EK, Crapo J, Hokanson JE, COPDGene Investigators. Pulmonary function reduction in diabetes with and without chronic obstructive pulmonary disease. Diabetes Care 2014. 37(2):389-395. [DOD] [CrossRef]
- Franssen FM, O'Donnell DE, Goossens GH, Blaak EE, Schols AM. Obesity and the lung: 5. Obesity and COPD. Thorax 2008. 63(12):1110-1117. [DOD] [CrossRef]
- Collins LC, Hoberty PD, Walker JF, Fletcher EC, Peiris AN. The effect of body fat distribution on pulmonary function tests. Chest 1995. 107(5):1298-1302. [DOD] [CrossRef]
- Bolton CE, Evans M, Ionescu AA, Edwards SM, Morris RH, Dunseath G, Luzio SD, Owens DR, Shale DJ. Insulin resistance and inflammation - a further systemic complication of COPD. COPD 2007. 4(2):121-126. [DOD] [CrossRef]
- Shaaban R, Kony S, Driss F, Leynaert B, Soussan D, Pin I, Neukirch F, Zureik M. Change in C-reactive protein levels and FEV1 decline: a longitudinal population-based study. Respir Med 2006. 100(12):2112-2120. [DOD] [CrossRef]
- Faber DR, van der Graaf Y, Westerink J, Visseren FL. Increased visceral adipose tissue mass is associated with increased C-reactive protein in patients with manifest vascular diseases. Atherosclerosis 2010. 212:274-280. [DOD] [CrossRef]
- Obata Y, Yamada Y, Takahi Y, Baden MY, Saisho K, Tamba S, Yamamoto K, Umeda M, Furubayashi A, Matsuzawa Y. Relationship between serum adiponectin levels and age in healthy subjects and patients with type 2 diabetes. Clin Endocrinol (Oxf) 2013. 79(2):204-210. [DOD] [CrossRef]
- Raghu G, Collard HR, Egan JJ, Martinez FJ, Behr J, Brown KK, Colby TV, Cordier JF, Flaherty KR, Lasky JA, et al. ATS/ERS/JRS/ALAT Committee on Idiopathic Pulmonary Fibrosis. An official ATS/ERS/JRS/ALAT statement: idiopathic pulmonary fibrosis: evidence-based guidelines for diagnosis and management. Am J Respir Crit Care Med 2011. 183(6):788-824. [DOD] [CrossRef]
- Iwai K, Mori T, Yamada N, Yamaguchi M, Hosoda Y. Idiopathic pulmonary fibrosis: epidemiologic approaches to occupational exposure. Am J Respir Crit Care Med 1994. 150:670-675. [DOD] [CrossRef]
- Raghu G, Weycker D, Edelsberg J, Bradford WZ, Oster G. Incidence and prevalence of idiopathic pulmonary fibrosis. Am J Respir Crit Care Med 2006. 174(7):810-816. [DOD] [CrossRef]
- Kim YJ, Park JW, Kyung SY, Lee SP, Chung MP, Kim YH, Lee JH, Kim YC, Ryu JS, Lee HL, et al. Clinical characteristics of idiopathic pulmonary fibrosis patients with diabetes mellitus: the national survey in Korea from 2003 to 2007. J Korean Med Sci 2012. 27(7):756-760. [DOD] [CrossRef]
- Gribbin J, Hubbard R, Smith C. Role of diabetes mellitus and gastro-oesophageal reflux in the aetiology of idiopathic pulmonary fibrosis. Respir Med 2009. 103(6):927-931. [DOD] [CrossRef]
- Enomoto T, Usuki J, Azuma A, Nakagawa T, Kudoh S. Diabetes mellitus may increase risk for idiopathic pulmonary fibrosis. Chest 2003. 123(6):2007-2011. [DOD] [CrossRef]
- Oldham JM, Collard HR. Comorbid conditions in idiopathic pulmonary fibrosis: recognition and management. Frontiers in Medicine 2017. 4:123. [DOD] [CrossRef]
- Sun XM, Tan JC, Zhu Y, Lin L. Association between diabetes mellitus and gastroesophageal reflux disease: a meta-analysis. World J Gastroenterol 2015. 21(10):3085-3092. [DOD] [CrossRef]
- Raghu G, Freudenberger TD, Yang S, Curtis JR, Spada C, Hayes J, Sillery JK, Pope CE II, Pellegrini CA. High prevalence of abnormal acid gastro-oesophageal reflux in idiopathic pulmonary fibrosis. Eur Respir J 2006. 27:136-142. [DOD] [CrossRef]
- Centers for Disease Control and Prevention. National Diabetes Statistics Report: Estimates of Diabetes and Its Burden in the United States. Atlanta, GA, USA. U.S. Department of Health and Human Services, 2014. [DOD]
- Pugh ME, Robbins IM, Rice TW, West J, Newman JH, Hemnes AR. Unrecognized glucose intolerance is common in pulmonary arterial hypertension. J Heart LungTransplant 2011. 30(8):904-911. [DOD]
- Lang IM, Simonneau G, Pepke-Zaba JW, Mayer E, Ambrož D, Blanco I, Torbicki A, Mellemkjaer S, Yaici A, Delcroix M. Factors associated with diagnosis and operability of chronic thromboembolic pulmonary hypertension. A case-control study. Thromb Haemost 2013. 110(1):83-91. [DOD]
- Abernethy AD, Stackhouse K, Hart S, Devendra G, Bashore TM, Dweik R, Krasuski RA. Impact of diabetes in patients with pulmonary hypertension. Pulm Circ 2015. 5(1):117-123. [DOD] [CrossRef]
- Mclaughlin V, Galie N, Barbera JA, Frost A, Ghofrani H. A comparison of characteristics and outcomes of patients with atypical and classical pulmonary arterial hypertension from the AMBITION trial. Proceedings of the Mini Symposium, ATS Conference, Denver, Colorado, USA, 2015. [DOD]
- Makarevich AE, Valevich VE, Pochtavtsev AU. Evaluation of pulmonary hypertension in COPD patients with diabetes. Adv Med Sci 2007. 52:265-272. [DOD]
- Gomez A, Bialostozky D, Zajarias A, Santos E, Palomar A, Martínez ML, Sandoval J. Right ventricular ischemia in patients with primary pulmonary hypertension. J Am Coll Cardiol 2001. 38(4):1137-1142. [DOD] [CrossRef]
- Benson L, Brittain EL, Pugh ME, Austin ED, Fox K, Wheeler L, Robbins IM, Hemnes AR. Impact of diabetes on survival and right ventricular compensation in pulmonary arterial hypertension. Pulm Circ 2014. 4(2):311-318. [DOD] [CrossRef]
- Belly MJ, Tiede H, Morty RE, Schulz R, Voswinckel R, Tanislav C, Olschewski H, Ghofrani HA, Seeger W, Reichenberger F. HbA1c in pulmonary arterial hypertension: a marker of prognostic relevance? J Heart Lung Transplant 2012. 31(10):1109-1114. [DOD]
- Di Paolo S, Gesualdo L, Ranieri E, Grandaliano G, Schena FP. High glucose concentration induces the overexpression of transforming growth factor-beta through the activation of a platelet-derived growth factor loop in human mesangial cells. Am J Pathol 1996. 149(6):2095-2106. [DOD]
- Arnold N, Koppula PR, Gul R, Luck C, Pulakat L. Regulation of cardiac expression of the diabetic marker microRNA miR-29. Plos One 2014. 9(7):e103284. [DOD] [CrossRef]
- Hua H, Goldberg HJ, Fantus IG, Whiteside CI. High glucose-enhanced mesangial cell extracellular signal-regulated protein kinase activation and alpha1(IV) collagen expression in response to endothelin-1: role of specific protein kinase C isozymes. Diabetes 2001. 50(10):2376-2383. [DOD] [CrossRef]
- Haffner SM, Lehto S, Rönnemaa T, Pyörälä K, Laakso M. Mortality from coronary heart disease in subjects with type 2 diabetes and in nondiabetic subjects with and without prior myocardial infarction. N Engl J Med 1998. 339(4):229-234. [DOD] [CrossRef]
- Lopez-Lopez JG, Moral-Sanz J, Frazziano G, Gomez-Villalobos MJ, Flores-Hernandez J, Monjaraz E, Cogolludo A, Perez-Vizcaino F. Diabetes induces pulmonary artery endothelial dysfunction by NADPH oxidase induction. Am J Physiol Lung Cell Mol Physiol 2008. 295(5):L727-L732. [DOD] [CrossRef]
- Williams SB, Goldfine AB, Timimi FK, Ting HH, Roddy MA, Simonson DC, Creager MA. Acute hyperglycemia attenuates endothelium-dependent vasodilation in humans in vivo. Circulation 1998. 97(17):1695-1701. [DOD] [CrossRef]
- Dewachter L, Dewachter C, Belhaj A. Insulin-like growth factor-1 contributes to the pulmonary artery smooth muscle cell proliferation in pulmonary arterial hypertension. Eur Resp J 2014. 44:58. [DOD]
- Hansmann G, Wagner RA, Schellong S, Perez VA, Urashima T, Wang L, Sheikh AY, Suen RS, Stewart DJ, Rabinovitch M. Pulmonary arterial hypertension is linked to insulin resistance and reversed by peroxisome proliferator-activated receptor-gamma activation. Circulation 2007. 115(10):1275-1284. [DOD] [CrossRef]
- Sona MF, Myung SK, Park K, Jargalsaikhan G. Type 1 diabetes mellitus and risk of cancer: a meta-analysis of observational studies. Jpn J Clin Oncol 2018. 48(5):426-433. [DOD] [CrossRef]
- Imai H, Kaira K, Mori K, Ono A, Akamatsu H, Matsumoto S, Taira T, Kenmotsu H, Harada H, Naito T, et al. Prognostic significance of diabetes mellitus in locally advanced non-small cell lung cancer. BMC Cancer 2015. 15:989. [DOD] [CrossRef]
- De Giorgio R, Barbara G, Cecconi A, Corinaldesi R, Mancini AM. Diabetes is associated with longer survival rates in patients with malignant tumors. Arch Intern Med 2000. 160(14):2217. [DOD] [CrossRef]
- Shieh SH, Probst JC, Sung FC, Tsai WC, Li YS, Chen CY. Decreased survival among lung cancer patients with co-morbid tuberculosis and diabetes. BMC Cancer 2012. 12:174. [DOD] [CrossRef]
- Yang WS, Yang Y, Yang G, Chow WH, Li HL, Gao YT, Ji BT, Rothman N, Zheng W, Shu XO, et al. Pre-existing type 2 diabetes and risk of lung cancer: a report from two prospective cohort studies of 133 024 Chinese adults in urban Shanghai. BMJ Open 2014. 4(7):e004875. [DOD] [CrossRef]
- Luo J, Hendryx M, Qi L, Ho GY, Margolis KL. Pre-existing diabetes and lung cancer prognosis. Br J Cancer 2016. 115(1):76-79. [DOD] [CrossRef]
- Inal A, Kaplan MA, Kucukoner M, Urakcı Z, Kılınc F, Isıkdogan A. Is diabetes mellitus a negative prognostic factor for the treatment of advanced non-small-cell lung cancer? Rev Port Pneumol 2014. 20(2):62-68. [DOD]
- Yu WS, Lee CY, Park SY, Suh JW, Narm KS, Kim DJ, Chung KY, Lee JG. Prognostic factors for resected non-small cell lung cancer in patients with type 2 diabetes mellitus. J Surg Oncol 2018. 117(5):985-993. [DOD] [CrossRef]
- Song H, Yu JM. Effect of diabetes mellitus on the development of radiation pneumonitis in patients with non-small cell lung cancer. Zhonghua Zhong Liu Za Zhi 2009. 31(1):45-47. [DOD]
- Zhou H, Cao K, Cao P, Jiang W. Impact of diabetes mellitus on clinicopathological factors and relation with radiation pneumonitis in 332 patients with lung cancer. Zhong Nan Da Xue Xue Bao Yi Xue Ban 2013. 38(2):138-141. [DOD]
- Yang X, Liu Y, Mani H, Olson J, Clawson G, Caruso C, Bruggeman R, Varlotto JM, Zander DS, Rassaei N. Biologic evaluation of diabetes and local recurrence in non-small cell lung cancer. Pathol Oncol Res 2017. 23(1):73-77. [DOD] [CrossRef]
- Karlin NJ, Amin SB, Buras MR, Kosiorek HE, Verona PM, Cook CB. Patient outcomes from lung cancer and diabetes mellitus: a matched case-control study. Future Sci OA 2017. 4(1):FSO248. [DOD] [CrossRef]
- Hanbali A, Al-Khasawneh K, Cole-Johnson C, Divine G, Ali H. Protective effect of diabetes against metastasis in patients with non-small cell lung cancer. Arch Intern Med 2007. 167(5):513. [DOD]
- Ikemura M, Nishikawa M, Kusamori K, Fukuoka M, Yamashita F, Hashida M. Pivotal role of oxidative stress in tumor metastasis under diabetic conditions in mice. J Control Release 2013. 170(2):191-197. [DOD] [CrossRef]
- Kang X, Kong F, Wu X, Ren Y, Wu S, Wu K, Jiang Z, Zhang W. High glucose promotes tumor invasion and increases metastasis-associated protein expression in human lung epithelial cells by upregulating heme oxygenase-1 via reactive oxygen species or the TGF-beta1/PI3K/Akt signaling pathway. Cell Physiol Biochem 2015. 35(3):1008-1022. [DOD] [CrossRef]
- Morss AS, Edelman ER. Glucose modulates basement membrane fibroblast growth factor-2 via alterations in endothelial cell permeability. J Biol Chem 2007. 282(19):14635-14644. [DOD] [CrossRef]
- Garcia-Jimenez C, Garcia-Martinez JM, Chocarro-Calvo A, De la Vieja A. A new link between diabetes and cancer: enhanced WNT/β-catenin signaling by high glucose. J Mol Endocrinol 2013. 52(1):R51-R66. [DOD] [CrossRef]
- Baker EH, Baines DL. Airway glucose homeostasis: a new target in the prevention and treatment of pulmonary infection. Chest 2018. 153(2):507-514. [DOD] [CrossRef]
- Xin WX, Fang L, Fang QL, Zheng XW, Ding HY, Huang P. Effect of hypoglycemic agents on survival outcomes of lung cancer patients with diabetes mellitus: a meta-analysis. Medicine (Baltimore) 2018. 97(9):e0035. [DOD] [CrossRef]
- Xu T, Li D, He Y, Zhang F, Qiao M, Chen Y. Prognostic value of metformin for non-small cell lung cancer patients with diabetes. World J Surg Oncol 2018. 16(1):60. [DOD] [CrossRef]
- Marrone KA, Zhou X, Forde PM, Purtell M, Brahmer JR, Hann CL, Kelly RJ, Coleman B, Gabrielson E, Rosner GL, et al. A randomized phase II study of metformin plus paclitaxel/carboplatin/bevacizumab in patients with chemotherapy-naïve advanced or metastatic nonsquamous non-small cell lung cancer. Oncologist 2018. 23(7):859-865. [DOD] [CrossRef]
- Wang J, Wang Y, Han J, Mei H, Yu D, Ding Q, Zhang T, Wu G, Peng G, Lin Z. Metformin attenuates radiation-induced pulmonary fibrosis in a murine model. Radiat Res 2017. 188(1):105-113. [DOD] [CrossRef]
- Choi SM, Jang AH, Kim H, Lee KH, Kim YW. Metformin reduces bleomycin-induced pulmonary fibrosis in mice. J Korean Med Sci 2016. 31(9):1419-1425. [DOD] [CrossRef]
- Vicentini M, Ballotari P, Giorgi Rossi P, Venturelli F, Sacchettini C, Greci M, Mangone L, Pezzarossi A, Manicardi V. Effect of different glucose-lowering therapies on cancer incidence in type 2 diabetes: an observational population-based study. Diabetes Res Clin Pract 2018. 143:398-408. [DOD] [CrossRef]
- Kajiwara C, Kusaka Y, Kimura S, Yamaguchi T, Nanjo Y, Ishii Y, Udono H, Standiford TJ, Tateda K. Metformin mediates protection against legionella pneumonia through activation of AMPK and mitochondrial reactive oxygen species. J Immunol 2018. 200(2):623-631. [DOD] [CrossRef]
- Patkee WR, Carr G, Baker EH, Baines DL, Garnett JP. Metformin prevents the effects of Pseudomonas aeruginosa on airway epithelial tight junctions and restricts hyperglycaemia-induced bacterial growth. J Cell Mol Med 2016. 20(4):758-764. [DOD] [CrossRef]
- Singhal A, Jie L, Kumar P, Hong GS, Leow MK, Paleja B, Tsenova L, Kurepina N, Chen J, Zolezzi F, et al. Metformin as adjunct antituberculosis therapy. Sci Transl Med 2014. 6(263):263ra159. [DOD] [CrossRef]
- Li L, Huang W, Li K, Zhang K, Lin C, Han R, Lu C, Wang Y, Chen H, Sun F, et al. Metformin attenuates gefitinib-induced exacerbation of pulmonary fibrosis by inhibition of TGF-beta signaling pathway. Oncotarget 2015. 6(41):43605-43619. [DOD]
- Sato N, Takasaka N, Yoshida M, Tsubouchi K, Minagawa S, Araya J, Saito N, Fujita Y, Kurita Y, Kobayashi K, et al. Metformin attenuates lung fibrosis development via NOX4 suppression. Respir Res 2016. 17(1):107. [DOD] [CrossRef]
- Li CY, Erickson SR, Wu CH. Metformin use and asthma outcomes among patients with concurrent asthma and diabetes. Respirology 2016. 21(7):1210-1218. [DOD] [CrossRef]
- Cui W, Zhang Z, Zhang P, Qu J, Zheng C, Mo X, Zhou W, Xu L, Yao H, Gao J. Nrf2 attenuates inflammatory response in COPD/emphysema: crosstalk with Wnt3a/beta-catenin and AMPK pathways. J Cell Mol Med 2018. 22(7):3514-3525. [DOD] [CrossRef]
- Jiang J, Ren HY, Geng GJ, Mi YJ, Liu Y, Li N, Yang SY, Shen DY. Oncogenic activity of insulin in the development of non-small cell lung carcinoma. Oncol Lett 2018. 15(1):447-452. [DOD]
- Liu J, Jia Y, Jia L, Li T, Yang L, Zhang G. MicroRNA-615-3p inhibits the tumor growth and metastasis of NSCLC via inhibiting IGF2. Oncol Res 2018. In press. [DOD]
- Zhou J, Wang J, Zeng Y, Zhang X, Hu Q, Zheng J, Chen B, Xie B, Zhang WM. Implication of epithelial-mesenchymal transition in IGF1R-induced resistance to EGFR-TKIs in advanced non-small cell lung cancer. Oncotarget 2015. 6(42):44332-44345. [DOD]
- Jansen C, Speck M, Greineisen WE, Maaetoft-Udsen K, Cordasco E, Shimoda LM, Stokes AJ, Turner H. Transcriptional and functional plasticity induced by chronic insulin exposure in a mast cell-like basophilic leukemia cell model. J Immunobiol 2017. 2(4):135. [DOD]
- Nie Z, Jacoby DB, Fryer AD. Hyperinsulinemia potentiates airway responsiveness to parasympathetic nerve stimulation in obese rats. Am J Respir Cell Mol Biol 2014. 51(2):251-261. [DOD]
- Dekkers BG, Schaafsma D, Tran T, Zaagsma J, Meurs H. Insulin-induced laminin expression promotes a hypercontractile airway smooth muscle phenotype. Am J Respir Cell Mol Biol 2009. 41(4):494-504. [DOD] [CrossRef]
- Viardot A, Grey ST, Mackay F, Chisholm D. Potential antiinflammatory role of insulin via the preferential polarization of effector T cells toward a T helper 2 phenotype. Endocrinology 2007. 148(1):346-353. [DOD] [CrossRef]
- Chen CZ, Hsu CH, Li CY, Hsiue TR. Insulin use increases risk of asthma but metformin use reduces the risk in patients with diabetes in a Taiwanese population cohort. J Asthma 2017. 54(10):1019-1025. [DOD] [CrossRef]
- Ruan W, Ying K. Abnormal expression of IGF-binding proteins, an initiating event in idiopathic pulmonary fibrosis? Pathol Res Pract 2010. 206(8):537-543. [DOD]
- Xu J, Zhu YT, Wang GZ, Han D, Wu YY, Zhang DX, Liu Y, Zhang YH, Xie XM, Li SJ, et al. The PPARgamma agonist, rosiglitazone, attenuates airway inflammation and remodeling via heme oxygenase-1 in murine model of asthma. Acta Pharmacol Sin 2015. 36(2):171-178. [DOD] [CrossRef]
- Shen Y, Chen L, Wang T, Wen F. PPARgamma as a potential target to treat airway mucus hypersecretion in chronic airway inflammatory diseases. PPAR Res 2012. 2012:256874. [DOD] [CrossRef]
- Grommes J, Mörgelin M, Soehnlein O. Pioglitazone attenuates endotoxin-induced acute lung injury by reducing neutrophil recruitment. Eur Respir J 2012. 40(2):416-423. [DOD] [CrossRef]
- Ito K, Shimada J, Kato D, Toda S, Takagi T, Naito Y, Yoshikawa T, Kitamura N. Protective effects of preischemic treatment with pioglitazone, a peroxisome proliferator-activated receptor-gamma ligand, on lung ischemia-reperfusion injury in rats. Eur J Cardiothorac Surg 2004. 25(4):530-536. [DOD] [CrossRef]
- Sime PJ. The antifibrogenic potential of PPARgamma ligands in pulmonary fibrosis. J Investig Med 2008. 56(2):534-538. [DOD] [CrossRef]
- Aoki Y, Maeno T, Aoyagi K, Ueno M, Aoki F, Aoki N, Nakagawa J, Sando Y, Shimizu Y, Suga T, et al. Pioglitazone, a peroxisome proliferator-activated receptor gamma ligand, suppresses bleomycin-induced acute lung injury and fibrosis. Respiration 2009. 77(3):311-319. [DOD] [CrossRef]
- El-Naa MM, El-Refaei MF, Nasif WA, Abduljawad SH, El-Brairy AI, El-Readi MZ. In-vivo antioxidant and anti-inflammatory activity of rosiglitazone, a peroxisome proliferator-activated receptor-gamma (PPAR-γ) agonists in animal model of bronchial asthma. J Pharm Pharmacol 2015. 67(10):1421-1430. [DOD] [CrossRef]
- Chen DL, Huang HJ, Byers DE, Shifren A, Belikoff B, Engle JT, Arentson E, Kemp D, Phillips S, Scherrer DE, et al. The peroxisome proliferator-activated receptor agonist pioglitazone and 5-lipoxygenase inhibitor zileuton have no effect on lung inflammation in healthy volunteers by positron emission tomography in a single-blind placebo-controlled cohort study. Plos One 2018. 13(2):e0191783. [DOD] [CrossRef]
- Nazim UM, Moon JH, Lee YJ, Seol JW, Park SY. PPARgamma activation by troglitazone enhances human lung cancer cells to TRAIL-induced apoptosis via autophagy flux. Oncotarget 2017. 8(16):26819-26831. [DOD] [CrossRef]
- Reddy AT, Lakshmi SP, Reddy RC. PPARgamma as a novel therapeutic target in lung cancer. PPAR Res 2016. 2016:8972570. [DOD]
- Ni J, Zhou LL, Ding L, Zhao X, Cao H, Fan F, Li H, Lou R, Du Y, Dong S, et al. PPARgamma agonist efatutazone and gefitinib synergistically inhibit the proliferation of EGFR-TKI-resistant lung adenocarcinoma cells via the PPARgamma/PTEN/Akt pathway. Exp Cell Res 2017. 361(2):246-256. [DOD] [CrossRef]
- Nieto-Fontarigo JJ, Gonzalez-Barcala FJ, San Jose E, Arias P, Nogueira M, Salgado FJ. CD26 and asthma: a comprehensive review. Clin Rev Allergy Immunol 2016. In press. [DOD]
- Colice G, Price D, Gerhardsson de Verdier M, Rabon-Stith K, Ambrose C, Cappell K, Irwin DE, Juneau P, Vlahiotis A. The effect of DPP-4 inhibitors on asthma control: an administrative database study to evaluate a potential pathophysiological relationship. Pragmat Obs Res 2017. 8:231-240. [DOD]
- Chang CH, Lin JW, Wu LC, Lai MS, Chuang LM. Oral insulin secretagogues, insulin, and cancer risk in type 2 diabetes mellitus. J Clin Endocrinol Metab 2012. 97(7):E1170-E1175. [DOD] [CrossRef]
- Wu Y, Liu HB, Shi XF, Song Y. Conventional hypoglycaemic agents and the risk of lung cancer in patients with diabetes: a meta-analysis. Plos One 2014. 9(6):e99577. [DOD] [CrossRef]
- Nazim UM, Moon JH, Lee YJ, Seol JW, Kim YJ, Park SY. Glipizide sensitizes lung cancer cells to TRAIL-induced apoptosis via Akt/mTOR/autophagy pathways. Oncotarget 2017. 8(59):100021-100033. [DOD] [CrossRef]
- Cui W, Zhang S, Cai Z, Hu X, Zhang R, Wang Y, Li N, Chen Z, Zhang G. The antidiabetic agent glibenclamide protects airway hyperresponsiveness and inflammation in mice. Inflammation 2015. 38(2):835-845. [DOD] [CrossRef]
- Bankers-Fulbright JL, Kephart GM, Loegering DA, Bradford AL, Okada S, Kita H, Gleich GJ. Sulfonylureas inhibit cytokine-induced eosinophil survival and activation. J Immunol 1998. 160(11):5546-5553. [DOD]
- Wei Y, Mojsov S. Tissue specific expression of different human receptor types for pituitary adenylate cyclase activating polypeptide and vasoactive intestinal polypeptide: implications of their role in human physiology. J Neuroendocrinol 1996. 8(11):811-817. [DOD] [CrossRef]
- Romani-Perez M, Outeirino-Iglesias V, Gil-Lozano M, Gonzalez-Matias LC, Mallo F, Vigo E. Pulmonary GLP-1 receptor increases at birth and exogenous GLP-1 receptor agonists augmented surfactant-protein levels in litters from normal and nitrofen-treated pregnant rats. Endocrinology 2013. 154(3):1144-1155. [DOD] [CrossRef]
- Viby NE, Isidor MS, Buggeskov KB, Poulsen SS, Hansen JB, Kissow H. Glucagon-like peptide-1 (GLP-1) reduces mortality and improves lung function in a model of experimental obstructive lung disease in female mice. Endocrinology 2013. 154(12):4503-4511. [DOD] [CrossRef]
- Arakawa M, Mita T, Azuma K, Ebato C, Goto H, Nomiyama T, Fujitani Y, Hirose T, Kawamori R, Watada H. Inhibition of monocyte adhesion to endothelial cells and attenuation of atherosclerotic lesion by a glucagon-like peptide-1 receptor agonist, exendin-4. Diabetes 2010. 59(4):1030-1037. [DOD] [CrossRef]
- Iwai T, Ito S, Tanimitsu K, Udagawa S, Oka J. Glucagon-like peptide-1 inhibits LPS-induced IL-1beta production in cultured rat astrocytes. Neurosci Res 2006. 55:352-360. [DOD] [CrossRef]
- Sun YH, He L, Yan MY, Zhao RQ, Li B, Wang F, Yang Y, Yu HP. Overexpression of GLP-1 receptors suppresses proliferation and cytokine release by airway smooth muscle cells of patients with chronic obstructive pulmonary disease via activation of ABCA1. Mol Med Rep 2017. 16(1):929-936. [DOD] [CrossRef]
- Baptist G. The cardiovascular benefits associated with the use of sodium-glucose cotransporter 2 inhibitors - real-world data. Eur Endocrinol 2018. 14(1):17-23. [DOD] [CrossRef]
- Han Y, Cho YE, Ayon R, Guo R, Youssef KD, Pan M, Dai A, Yuan JX, Makino A. SGLT inhibitors attenuate NO-dependent vascular relaxation in the pulmonary artery but not in the coronary artery. Am J Physiol Lung Cell Mol Physiol 2015. 309(9):L1027-L1036. [DOD] [CrossRef]
|