Review
Rev Diabet Stud,
2011,
8(3):323-338 |
DOI 10.1900/RDS.2011.8.323 |
The Role of Incretin Therapy at Different Stages of Diabetes
Simona Cernea
Diabetes, Nutrition and Metabolic Diseases Outpatient Unit, Emergency County Clinical Hospital, Targu Mures, Romania
Manuscript submitted June 27, 2011; resubmitted August 1, 2011; accepted August 10, 2011.
Keywords: type 2 diabetes, glycemic control, incretin, GLP-1, DPP-4, GIP, liraglutide, insulin
Abstract
The pathogenetic mechanisms causing type 2 diabetes are complex, and include a significant reduction of the incretin effect. In patients with type 2 diabetes, GLP-1 secretion may be impaired, while GIP secretion seems unaffected. In contrast, the insulinotropic activity of GIP is severely altered, whereas that of GLP-1 is maintained to a great extent. Better understanding of the role of incretin hormones in glucose homeostasis has led to the development of incretin-based therapies that complement and offer important advantages over previously used agents. Incretin-based agents have significant glucose-lowering effects, promote weight loss (or are weight-neutral), inhibit glucagon secretion while maintaining counter-regulatory mechanisms, exhibit cardiovascular benefits, and protect β-cells while possessing a low risk profile. At present, incretin-based therapies are most widely used as add on to metformin to provide sufficient glycemic control after metformin failure. However, they are also recommended as monotherapy early in the disease course, and later in triple combination. These agents may also be a promising therapeutic tool in prediabetic subjects. Therefore, a therapeutic algorithm is needed for their optimal application at different stages of diabetes, as suggested in this article.
Abbreviations: ADA - American Diabetes Association; BID - twice a day; BMI - body mass index; CI - confidence interval; DPP-4 - dipeptidyl peptidase-4; EASD - European Association for the Study of Diabetes; EMA - European Medicines Agency; FDA - Food and Drug Administration; GIP - glucose-dependent insulinotropic polypeptide; GLP-1 - glucagon-like peptide-1; GLP-1R - GLP-1 receptor; HbA1c - glycated hemoglobin; IFG - impaired fasting glucose; IGT - impaired glucose tolerance; NEFA - nonesterified fatty acid; QD - once a day; TZD - thiazolidinediones
Pathophysiological considerations
The core pathophysiological defects leading to type 2 diabetes include increased resistance to insulin action in peripheral tissues and inadequate insulin secretion caused by a progressive decline in β-cell function. It has become apparent that other factors are also involved in the natural history of the disease [1]. Additional mechanisms that further exacerbate the pathological pathways include incretin deficiency and/or resistance, hyperglucagonemia and increased hepatic sensitivity to glucagon, altered fat metabolism caused by insulin resistance in adipocytes, enhanced glucose reabsorption in the kidneys, and insulin resistance in the central nervous system resulting from neurotransmitter dysfunction [1]. Early in the course of the disease, pancreatic insulin secretion is increased in an attempt to compensate for insulin resistance. Later, β-cells fail to sustain increased secretory rates, which results in gradually declining insulin release and hyperglycemia. Chronically elevated glucose (and lipid) levels cause gluco-lipotoxicity, which in turn further amplifies β-cell failure (by causing de-differentiation of pancreatic β-cells, activation of stress response, accelerated apoptosis, and decreased proliferation) and aggravates insulin resistance (Figure 1) [2].
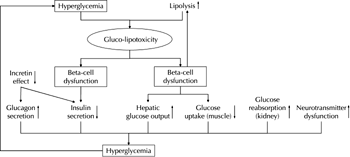 |
 |
Figure 1. Pathogenetic mechanisms leading to hyperglycemia and type 2 diabetes [1, 2]. |
|
An important role in the regulation of glucose homeostasis is played by incretins, which are gut-derived hormones released in response to nutrient ingestion (mainly glucose and fat). The two most important hormones found to mediate the "incretin effect" (that is, higher insulin release in response to an oral glucose challenge compared to an equal intravenous glucose load) are glucose-dependent insulinotropic polypeptide (GIP) and glucagon-like peptide-1 (GLP-1) [3]. These hormones elicit a vast range of effects, including stimulation of insulin secretion in a glucose-dependent manner, and it is estimated that up to 70% of the overall postprandial insulin response to glucose is mediated by them [4, 5].
During the development and progression of type 2 diabetes, signals from gut-derived factors are attenuated because of defective release of incretin hormones and/or resistance to their action [6]. The diminished incretin effect is a substantial contributor to insulin deficiency. Combined β-cell dysfunction and incretin deficiency is followed by hyperglycemia, which in turn further amplifies the impairment of incretin secretion and action, in part by downregulating their receptors [2, 7].
The significantly reduced incretin effect has been attributed to decreased secretion. Most of the studies quantifying GIP secretion in subjects with type 2 diabetes have reported that GIP levels are normal or even higher compared with healthy controls [8-10]. However, the insulinotropic effects of GIP have constantly been shown to be attenuated in diabetes, even at supraphysiological levels, implying that secretory defects are not the hallmark of the disease [11-13]. The underlying causes for the impairment of GIP responsiveness in diabetes are not completely understood, but several hypotheses have been proposed, including GIP-receptor mutations, hyperglycemia-associated receptor downregulation/desensitization, post-receptor defects of intracellular machinery, and reduced β-cell function and mass [14-16].
The other defect in the entero-insular axis in type 2 diabetes is related to GLP-1. A number of studies that evaluated GLP-1 secretion levels have indicated similar secretion levels in response to an oral nutrient load in subjects with and without diabetes. However, some reports have shown small reductions in (total and late postmeal) GLP-1 levels, especially in patients with long disease duration and poor metabolic control [8, 17, 18]. In contrast to GIP, the insulinotropic and glucagonostatic activity of GLP-1 is preserved to a high degree in patients with type 2 diabetes. The same applies to its ability to decelerate gastric emptying, although higher levels are needed to maintain physiological activity [12, 19-22]. Several factors, which influence GLP-1 secretory response, have been identified; these include older age, higher weight/BMI, glucagon concentrations, and fasting NEFA [23]. The mechanisms that lead to a glucagon-induced suppression of GLP-1 are still unknown.
To recapitulate, the secretion of GLP-1 is impaired in most patients with diabetes, while the secretion of GIP appears to be unaffected. In contrast, the insulinotropic activity of GIP is severely altered, whereas that of GLP-1 is maintained to a great extent. The two incretins are released independently of each other and have different abilities to stimulate the early- and late-phase insulin secretion which is explained by their distinct intracellular actions: GIP stimulates insulin release from readily available pools in β-cells, while GLP-1 accesses the reserve pools, stimulates insulin biosynthesis, and renders glucose-resistant β-cells more sensitive [9, 22].
Another explanation proposed for the impaired incretin effect is the reduction in overall β-cell function and mass, which occurs during the natural disease history and the defective secretory capacity of β-cells in response to incretin stimuli [6]. Some studies have indicated that the reduction in hyperglycemia partially reverses the impairment of GLP-1 and GIP actions and restores GLP-1 concentrations. This suggests that the diminished incretin effect is rather a consequence of the diabetic state and hyperglycemia, although a genetic component cannot be completely excluded [24].
To explore further the role of incretin release and action dysregulation in the pathophysiology of β-cell failure, studies evaluated GIP/GLP-1 secretion in the pre-diabetic state. The results are inconsistent so far. Some studies indicated modest impairments in GLP-1/GIP secretion, some found increased GIP levels (in association with hyperinsulinemia), and others found no alteration in incretin secretion [25-29]. Thus, while defects in incretin hormone secretion might be present in some subjects with impaired glucose tolerance (IGT), both IGT and diabetes can develop in the absence of alterations in incretin secretion. This reinforces the hypothesis that defective incretin release and action in patients with type 2 diabetes is an epiphenomenon of chronic hyperglycemia and not a primary cause [6].
It can thus be concluded that impairment in incretin activity contributes to the deterioration in glucose homeostasis (mainly in the postprandial phase) in patients with diabetes, but is unlikely to predispose to diabetes development.
Incretin-based therapies in the management of type 2 diabetes
With the development of new classes of drugs, treatment options became wider and the management of type 2 diabetes more complex. On the other hand, patients with diabetes have various clinical presentations, different courses of disease, and also different responses to therapeutic agents. When choosing the appropriate therapeutic strategy, several factors should be taken into consideration. Some of these factors are patient-specific (such as age, BMI/waist, duration of diabetes, co-morbidities), some are drug-specific (e.g. mechanisms of action, side effects), while others relate to the disease mechanisms.
Ideally, therapies should address early all pathophysiological disturbances to delay disease progression and to obtain long-lasting metabolic control. Current therapeutic algorithms use oral agents in a stepwise, additive manner when specific targets are not reached. This approach, however, does not prevent β-cell loss, nor does it assure sustainable glycemic control, and ultimately it leads to treatment failure [1, 30]. Also, the use of available agents is often impeded by their adverse effects (mainly hypoglycemia, weight gain, and edema) and/or the inability to meet certain requirements (such as optimal control of postprandial hyperglycemia) [30-32] (Table 1).
Table
1.
Possible treatment algorithm for type 2 diabetes therapy at various stages |
|
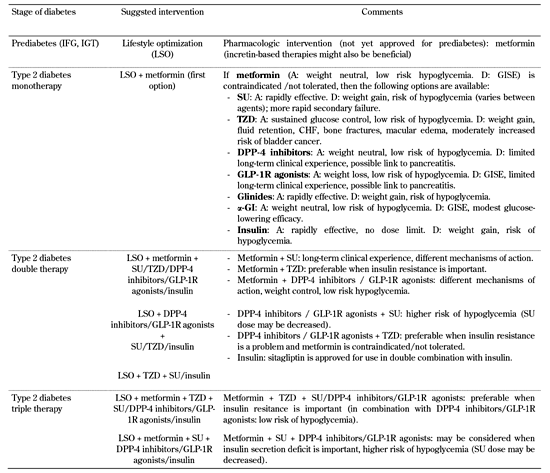 |
 |
Legend:
A: advantages. D: disadvantages. GISE: gastrointestinal side effects. SU: sulfonylureas. TZD: thiazolidindiones. CHF: congestive heart failure. α-GI: α-glucosidase inhibitors. |
|
Therefore, new drugs have been developed that target the core abnormalities of diabetes and have minimal side effects. A better understanding of the role of incretin hormones in maintaining glucose homeostasis has led to the development of two groups of incretin-based therapies: 1. GLP-1 receptor (GLP-1R) agonists, which are degradation-resistant synthetic/chemically-modified peptides that bind GLP-1 receptors and mimic the action of naturally occurring GLP-1 (incretin mimetics), and 2. dipeptidyl peptidase 4 (DPP-4) inhibitors, which, by inhibiting the enzyme, decrease the degradation of endogenous incretin hormones (both GLP-1 and GIP), and thus prolong their activity (incretin enhancers) [22].
The biological effects of incretins or incretin-based therapies have been reviewed in detail elsewhere [33, 34]. At the pancreatic level, incretins have pleiotropic actions. After binding to specific receptors on β-cells incretins promote the following effects:
1. Insulin gene transcription, biosynthesis, and determination of a glucose-dependent stimulation of insulin secretion (in conjunction with reduction of plasma glucagon and delayed gastric emptying, this results in a glucose-lowering effect).
2. β-cell survival and possibly β-cell proliferation/neogenesis.
3. Glucose-dependent inhibition of glucagon secretion [12, 35-37].
4. Improvement of β-cell responsiveness to glucose (possibly through upregulation of the biosynthesis of other β-cell products such as glucokinase and glucose transporters) [38].
Furthermore, GLP-1/GLP-1R agonists shift the dynamics of insulin secretion towards an earlier response and restore the biphasic profile [39].
In addition, a number of extrapancreatic actions have been described, some contributing to the glucoregulatory effects [40]. Incretins regulate feeding behavior by enhancing satiety/suppressing appetite and inhibiting caloric intake, while meal-stimulated gastric acid secretion and gastric emptying are inhibited in a dose-dependent fashion. The deceleration of gastric emptying is associated with a blunting of postprandial glucose excursions and insulin levels [41, 42]. Moreover, the effects of incretins on lipid profile are either neutral or slightly beneficial, and they also have cardioprotective effects (they lower blood pressure, ameliorate endothelial and myocardial function) [43]. Some data, mainly based on animal studies, suggest that they even improve insulin sensitivity, although this effect is not dominant [43-45]. Among other concomitant beneficial effects, of particular relevance is the reduced risk of (severe) hypoglycemia, due to the fact that the insulininotropic effect is strictly glucose-dependent, and because of the preservation of normal counter-regulatory mechanisms.
Incretin therapy in prediabetes: impaired glucose tolerance (IGT) and impaired fasting glucose (IFG)
Evidence exists that the period between the earliest abnormalities of glucose metabolism and the onset of diabetes is long and that a high proportion of individuals with prediabetes are likely to progress to diabetes or to remain in the abnormal glycemic state [46]. Therefore, various intervention strategies to prevent progression to overt disease have been sought. Questions have been raised whether these interventions, even if effective, truly prevent or just delay the disease. A definite answer would require long-term follow-up studies, but even in the second situation, a potential benefit could be envisaged, given the fact that life-time exposure to higher glycemic concentrations is shortened, and thus diabetes-related morbidity deferred [47].
Well-designed preventive trials have demonstrated that lifestyle modification and pharmacologic interventions that target hyperglycemia are effective in reducing the occurrence of diabetes in at-risk individuals [48-58]. Several clinical trials have indicated that intensive lifestyle interventions provide the greatest decrease in the incidence of diabetes [48-50]. Pharmacological agents (metformin, thiazolidinediones (TZD), acarbose, nateglinide, orlistat, ramipril, and valsartan) have been evaluated for the prevention of diabetes and cardiovascular disease in patients with IFG/IGT and some of them have been associated with slower progression to diabetes [51-58]. However, there are many issues (including cost-effectiveness) that need to be considered before medications are recommended for preventive purposes. The American Diabetes Association (ADA) consensus statement indicates that metformin is the only oral agent that should be used in subjects having IFG, IGT, and an additional risk factor [46]. However, the use of medication in IFG/IGT is not yet approved by regulatory agencies.
Evidence for the efficacy of incretin-based therapy in the prediabetic stage is minor. Some data suggest that obese individuals with IFG or IGT treated with exenatide along with lifestyle interventions for 24 weeks reverted to normal glucose tolerance [59]. In individuals with IFG, therapy with vildagliptin improved postprandial glucose levels after 6 weeks, but the effect was not maintained beyond the wash-out period, possibly because of the short duration of treatment [60]. Similarly, an eight-week treatment with sitagliptin did not change fasting or postprandial glucose levels in subjects with IFG, but again, it is not clear whether the duration of therapy was long enough for the effects to occur [61]. A twelve-week study, however, reported that IGT subjects treated with vildagliptin presented a decrease in peak and total glycemic excursions [62]. Thus, limited evidence regarding incretin-based therapy in prediabetes seems to point to some benefit, but it appears that the treatment needs to be of long duration. It is hypothesized that these agents might be used to prevent or delay the progression to overt diabetes, but long-term, large clinical trials are needed to evaluate this potential. It is however necessary to demonstrate first that incretin-based therapies improve glucose homeostasis (and maybe also β-cell function/mass) in prediabetic individuals.
Incretin therapy in the early stages of type 2 diabetes (monotherapy)
Current ADA, EASD, and other association guidelines for the management of type 2 diabetes advocate initiating lifestyle changes (to decrease weight and increase physical activity) and metformin early after diagnosis to achieve glycemic goals [31, 63-65]. Metformin is the preferred first therapy for most patients, barring contraindications, because of the extensive clinical experience available, its beneficial safety profile, and its cost-effectiveness. Recent data indicate that metformin also modulates components of the incretin axis; it increases GLP-1 concentration by enhancing preproglucagon expression, and increases expression of the GLP-1 receptor [66, 67].
However, the choice of the glucose-lowering drug should be individualized, and specific patient characteristics, problems, and disease-specific factors should also be taken into account, beside those related to the drug itself. When considering drugs, not only the glucose-lowering effects should be taken into account, but also other advantages and disadvantages should be considered within the limits of approved indications (Table 1) [32, 68].
Because of limited study data and clinical experience, guidelines currently do not recommend incretin-based therapies as first option for monotherapy in newly diagnosed diabetes. However, in certain situations (e.g. when metformin is contraindicated or not tolerated, and when hypoglycemia or weight gain constitute a problem), they should be considered as a treatment option. GLP-1R agonists have certain advantages such as low risk of hypoglycemia, favorable weight effects allowing weight loss, potential cardiovascular benefits, and protective effects on β-cells. They also have proven efficacy in terms of reducing fasting and postprandial glucose concentration and glycated hemoglobin (HbA1c) [69, 70]. A recent meta-analysis indicated that compared with placebo, patients showed the following overall reductions in HbA1c when treated with different agents [70]:
1. Liraglutide -1.03% (95% CI: -1.16 to -0.90, p < 0.001).
2. Exenatide -0.75% (95% CI: -0.83 to -0.67, p < 0.001).
3. Sitagliptin -0.79% (95% CI: -0.93 to -0.65, p < 0.001).
4. Vildagliptin -0.67% (95% CI: -0.83 to -0.52, p < 0.001).
Another meta-analysis combined data from randomized controlled trials (with at least a 12-week duration) that used a GLP-1R agonist or a DPP-4 inhibitor. It showed that a higher proportion of patients treated with an incretin-based therapeutic agent achieved target goals of HbA1c < 7.0% compared to placebo/standard treatment groups at the end of the study period [69]. Table 2 summarizes the efficacy data in clinical trials that evaluated GLP-1R agonists and Tables 3 and 4 the data on DPP-4 inhibitors in monotherapy and combination therapy.
Table
2.
Summary of efficacy data in randomized controlled trials with GLP-1R agonists |
|
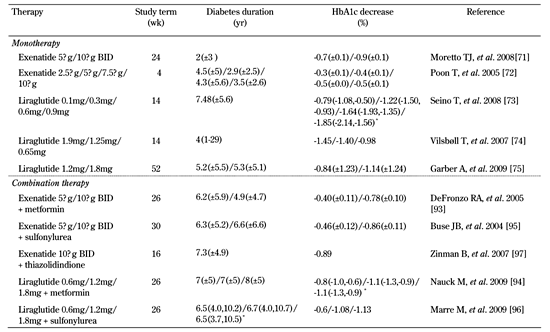 |
 |
Legend:
Data for diabetes duration are mean ± SD. BID: twice daily. * Odds ratio and 95% CI. |
|
Table
3.
Summary of efficacy data in randomized controlled trials with DPP-4 inhibitors monotherapy |
|
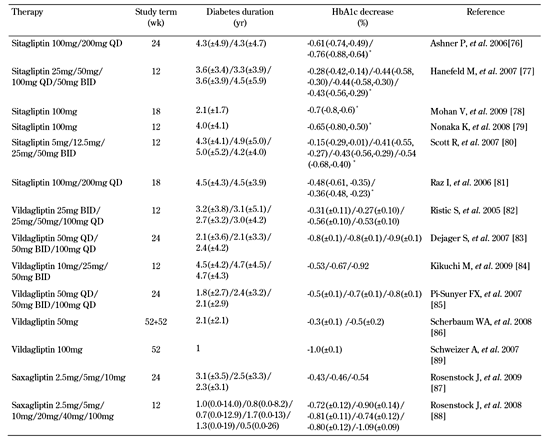 |
 |
Legend:
Data for diabetes duration are mean ± SD. BID: twice daily. QD: once daily. * Odds ratio and 95% CI. |
|
Table
4.
Summary of efficacy data in randomized controlled trials with DPP-4 inhibitors combination therapy |
|
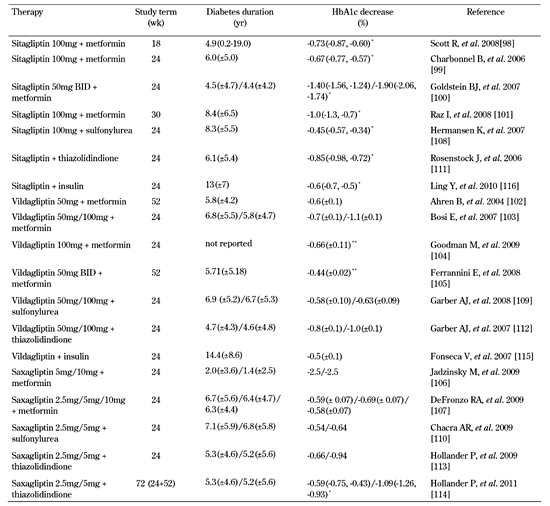 |
 |
Legend:
Data for diabetes duration are mean ± SD. BID: twice daily. QD: once daily. * Odds ratio and 95% CI. ** mean ± SE. |
|
Data from clinical trials indicate that, when used as monotherapy, exenatide 5 µg/10 µg BID showed a significant reduction in mean HbA1c compared with placebo (in the range of -0.4% to -0.9%), while various doses of liraglutide (from 0.1 to 1.9 mg QD) caused a dose-depended decrease in HbA1c ranging from -0.79% to -1.85% [71-74]. In comparison to a sulfonylurea (i.e. glimepiride), 1.2 mg/1.8 mg of liraglutide in monotherapy significantly lowered mean HbA1c by 0.84%/1.14% [75].
Even more clinical studies evaluated the effect of DPP-4 inhibitors as single therapeutic agents on glycemic control. Various doses of sitagliptin (starting from 5 mg BID to 200 mg QD), given in subjects not controlled by lifestyle interventions alone, determined a dose-dependent decrease in HbA1c ranging from -0.15% to -0.76% [76-81]. Sitagliptin 100 mg QD (the regimen used in clinical practice) lowered mean HbA1c by 0.44% to 0.7% [75-78, 80]. Monotherapy with vildagliptin in doses evaluated in clinical trials ranging from 10 mg BID to 100 mg QD achieved a 0.2% to 0.92% reduction in HbA1c versus placebo at study end [82-86]. Intervention with saxagliptin as monotherapy at different doses (2.5 mg to 100 mg) was associated with a significant decrease in HbA1c ranging from -0.43% to -1.09% compared with placebo [87, 88]. Compared with metformin, 100 mg vildagliptin in monotherapy significantly reduced mean HbA1c by 1.0% from baseline, but statistical non-inferiority to metformin 1000 mg twice daily has not been demonstrated [89].
At present, only sitagliptin (100 mg QD) is approved for use as monotherapy by both the European Medicines Agency (EMA) and US Food and Drug Administration (FDA). Exenatide (5 µg/10 µg BID) and saxagliptin (2.5 mg/5 mg QD) are approved as monotherapy only by the FDA. Vildagliptin is approved by the EMA only.
Incretin therapy in more advanced stages of type 2 diabetes (double or triple combination)
In the second step, treatment intensification with incretin mimetics and DPP-4 inhibitors is appropriate when one drug alone is not sufficient to achieve glycemic goals. Basically, there are four options, namely adding incretin mimetics to i) metformin, ii) pioglitazone (the only available drug of the TZD class at present), iii) a sulfonylurea, or iv) insulin.
When used in association with metformin or pioglitazone, the main advantages of incretins are pathophysiological. These two drugs are insulin sensitizers (in liver, muscle, and adipose tissues in the case of TZDs), while incretins target different pathological mechanisms (as discussed above), and thus potentiate the glucose-lowering action.
When combined with metformin, the key benefits of incretins are avoidance of hypoglycemia and better weight control. The addition of DPP-4 inhibitors or incretin mimetics to metformin is of particular benefit in patients who need an increase in endogenous insulin secretion, but who would be at high risk for hypoglycemia from sulfonylureas. Both incretins and TZDs are supposed to have beneficial effects on β-cells by preserving and improving their function and possibly increasing β-cell mass [90]. Some data suggest that metformin has a protective effect on β-cells (indirectly by ameliorating gluco- and lipotoxicity), but a direct positive effect of metfomin on β-cells is not generally accepted [90].
Sulfonylureas have been associated with a progressive decline in β-cell function and a concomitant loss of glycemic control [1, 90]. Sulfonylureas are more problematic when used in combination with incretins because of the possible occurrence of glucose-independent stimulation of insulin secretion that increases the risk of hypoglycemia [91, 92]. Hypoglycemic episodes can be minimized by reducing the sulfonylurea dose on incretin initiation, but this approach may also decrease treatment effectiveness.
The complementary effect of incretin-based therapies and insulin on fasting and postprandial glucose control provides a rationale for association of these agents in the management of type 2 diabetes.
In clinical trials, exenatide 5 µg (10 µg) BID in association with metformin decreased mean HbA1c by 0.4% (0.8%), while liraglutide induced a decrease of 0.7% to 1.0% (for doses ranging from 0.6 mg to 1.8 mg) [93, 94]. In combination with a sulphonylurea, the mean HbA1c change was -0.46% (-0.86%) for 5 µg (10 µg) of exenatide BID, and -0.6% to -1.1% for doses ranging from 0.6 mg to 1.8 mg of liraglutide [95, 96]. Mean HbA1c was reduced by 0.89% when 10 µg BID exenatide was added to a thiazolidindione (± metformin) [97].
Several studies evaluated the effect on HbA1c when sitagliptin was added to metformin. They showed a decrease of 0.66% to 1.9% [98-101]. Vildagliptin in combination with metformin reduced mean HbA1c by 0.44%-0.9%, while saxagliptin combined with metformin caused a decrease of 0.58% to 2.5% (for doses between 2.5 mg and 10 mg) [102-107]. In association with a sulfonylurea, sitagliptin 100 mg decreased HbA1c by 0.3%, vildagliptin 50 mg (100 mg) by 0.58% (0.63%), and saxagliptin by 0.54%-0.64% [108-110]. Clinical studies indicated that, in association with pioglitazone, sitagliptin 100 mg lowered HbA1c by 0.85%, vildagliptin 50 mg (100 mg) by 0.8% (1.0%), and saxagliptin 2.5 mg (5 mg) combined with a TZD by 0.59% (1.09%) [111-114]. Combination of vildagliptin with insulin (intermediate- or long-acting insulin plus prandial insulin) yielded a significant reduction in HbA1c (-0.5%) compared with placebo [115]. Similarly, the addition of sitagliptin to ongoing insulin therapy (with or without metformin) significantly reduced HbA1c by 0.6% from baseline compared with placebo, regardless of the type of insulin (long-acting, intermediate acting or premixed) [116].
Liraglutide is approved for use in association with metfomin or a sulfonylurea, exenatide, vildagliptin, and saxagliptin in combination with metfomin or a sulfonylurea or TZD. Sitagliptin can be used with metformin, a sulfonylurea, TZD or insulin. Currently, there are combinations of drugs available (vildagliptin + metformin approved by the EMA, saxagliptin + metformin approved by the FDA, and sitagliptin + metfomin approved by both agencies), which have the potential to increase treatment compliance.
At the third stage of treatment intensification, a triple drug combination may be considered for patients that do not achieve adequate glycemic control on double therapy, and when insulin treatment is not the preferred choice. At this stage, the best alternative could be to associate incretin-based agents with metformin and a TZD, especially when insulin resistance is the main concern. Exenatide, liraglutide, and sitagliptin are approved for use in triple combination with metformin and sulfonylurea/TZD.
In clinical studies, when GLP-1R agonists were used in triple therapy (with TZD plus metformin or with a sulphonylurea plus metformin) HbA1c decreased by 0.55%-1.5% [117-121]. A similar decrease (-0.89%) was observed for sitagliptin in combination with two other oral agents (metformin plus sulphonylurea) versus placebo [108]. Several studies tested incretin mimetics against insulin. They found that exenatide provided similar glycemic control, and liraglutide was slightly superior to insulin glargine in terms of HbA1c reduction [118-120, 122-125]. The treatment with GLP-1R agonists was associated with significant weight loss, and there was no significant difference between treatment groups in the incidence of hypoglycemic episodes [118-120, 122-125]. However, one study, including subjects in a more advanced stage of diabetes (with high HbA1c values at baseline and a long duration of diabetes), reported that significantly more patients achieved glycemic goals when treated with premixed insulin than with exenatide [126]. This implies that incretin-based therapy should be initiated at a stage when there is sufficient β-cell function for the drugs to be effective. Therefore, at the third stage of therapy intensification, various factors should be considered to decide whether insulin initiation or triple therapy would be the best choice.
GLP-1R agonists or DPP-4 inhibitors?
Several aspects, apart from their mechanism of action, distinguish the two drug classes, GLP-1R agonists and DPP-4 inhibitors (Table 5). These aspects might help clinicians to identify which patients benefit most from intervention with a drug from which class. The main differences are:
1. GLP-1R agonists are given by subcutaneous injection, while DPP-4 inhibitors are administered as oral tablets. In certain situations, the route of administration might be of significance, as regarding the convenience of use and adherence.
2. GLP-1R agonists are associated with significant and sustained weight loss, while DPP-4 inhibitors tend to be weight-neutral.
3. Therapy with GLP-1R agonists is associated with higher incidence of adverse gastrointestinal effects, particularly nausea, while infections seem to be more frequent with DPP-4 inhibitors.
4. GLP-1R agonists are generally associated with a slightly more robust glucose-lowering efficacy, as the mean HbA1c reductions are more pronounced (0.75%-1.03% with GLP-1R agonists compared to 0.67%-0.79% with DPP-4 inhibitors) [70]. Studies that directly compared exenatide or liraglutide with sitagliptin supported this assumption by demonstrating greater lowering of mean HbA1c (mean treatment differences for 1.8 mg (1.2 mg) liraglutide versus 100 mg sitagliptin were -0.60% (-0.34%), and -0.6% for 2 mg exenatide LAR versus 100 mg sitagliptin) [125, 126]. Also, some studies have shown that exenatide lowered 2-hour postprandial glucose levels more effectively, and caused significantly lower average 24-hour glucose, than sitagliptin [127, 128].
Table
5.
Clinical characteristics of GLP-1 receptor agonists and DPP-4 inhibitors [33, 66, 124] |
|
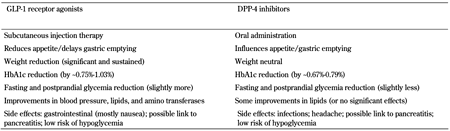 |
 |
The differences between incretin mimetics and incretin enhancers may be explained, at least in part, by higher circulating levels of the active agents obtained with GLP-1R agonists and possibly stronger receptor stimulation. Lower concentrations of endogenous GLP-1 are achieved after DPP-4 inhibition. Because of the progressive loss of GLP-1 secretion at more advanced stages of diabetes, it has been suggested that a replacement therapy using GLP-1R agonists is preferable over DPP-4 inhibitor treatment. DPP-4 inhibitors may not sufficiently lower blood glucose at later stages because diminished GLP-1 secretion [129-131]. However, studies that included patients with longer duration of diabetes treated with an incretin enhancer have shown efficacy in controlling blood glucose. On the other hand, there are no long-term longitudinal studies available at present that follow up the pattern of GLP-1 secretion during the natural history of the disease, and define the time-point and markers predicting the lack of response to DPP-4 inhibitors [23].
Conclusions and future directions
Incretin-based therapy complements and brings important and unique advantages to the therapeutic spectrum for type 2 diabetes. It addresses underlying pathophysiological abnormalities associated with the disease that are not targeted by other drugs, and has significant glucose-lowering and extraglycemic effects. There seems to be a consensus that a treatment algorithm based on pathophysiology is desirable. Thus, selecting (a combination of) drugs from classes that target various disease mechanisms would provide durable results in terms of metabolic control and would benefit β-cell function [1, 32]. Therefore, an early association between metformin ± pioglitazone (which increase insulin sensitivity, reduce hepatic glucose production (both drugs), inhibit lipolysis, and protect β-cells (TZD)) with an insulinotropic agent is reasonable.
A combination of sulfonylureas and DPP-4 inhibitors (or GLP-1R agonists) is beneficial early in the course of the disease. This is because sulfonylureas are associated with the progressive loss of metabolic control (after an initial decrease of HbA1c), which is due to the progressive loss of β-cells associated with their use. The combination with DPP-4 inhibitors (and GLP-1R agonists) would aid by improving β-cell function and maintain durability of glycemic control. Another benefit of adding incretin-based therapies is that they stimulate insulin secretion in a glucose-dependent manner. Therefore, they are associated with less hypoglycemia and weight gain than sulfonylureas. Finally, incretins target an additional pathological mechanism (hyperglucagonemia), in contrast to sulfonylureas. Therefore, even if these drugs are more expensive than sulfonylureas, it is easy to conclude that, in the future, incretin-based therapies should be used as insulinotropic agents on a larger scale and early in the disease course, because of their numerous advantages over sulfonylureas.
However, several points related to therapy with an incretin mimetic/enhancer still need to be evaluated and clarified:
1. When is the best moment to start incretin-based therapies, in the early or later stages of the disease? At present, these drugs are most likely to be used in addition to metformin, when monotherapy does not provide sufficient glycemic control. However, data from preclinical studies, indicating favorable effects on β-cell function, suggest that early intervention is more beneficial, but this needs to be proven by clinical trials. If the protective effect on β-cell health is assured, which might have disease-modifying potential by delaying the onset or slowing the progression of diabetes, then the current therapeutic algorithm may undergo changes.
2. Which drug (GLP-1R agonist or DPP-4 inhibitor) should be used at which stage? According to some suggestions, it is better to use DPP-4 inhibitors at an earlier stage of disease (when there is enough endogenous incretin secretion), while at later stages, a replacement therapy (with a GLP-1R agonist) is more efficient. However, this hypothesis needs validation by well-designed clinical trials.
3. Is there any marker that could predict a positive response to therapy? Identification of such markers would help to define the specific patient profiles that would be most likely to benefit from the therapy. It would also help to address patients’ needs more appropriately.
4. What is the impact of incretin-based therapy on long-term glycemic exposure and diabetic complications? Studies that could prove definite positive clinical outcomes (i.e. prevention or even improvement of chronic complications) will certainly help to improve diabetes management strategies.
There is evidence that not all patients with type 2 diabetes benefit equally from current treatment guidelines. Answers to the abovementioned and other questions will be helpful to characterize an efficient and individualized therapeutic approach for improving care.
Disclosures: The author reports no conflict of interests.
References
- DeFronzo RA. Current issues in the treatment of type 2 diabetes. Overview of newer agents: where treatment is going. Am J Med 2010. 123:S38-S48. [DOD] [CrossRef]
- Wajchenberg BL. Beta-cell failure in diabetes and preservation by clinical treatment. Endocr Rev 2007. 28:187-218. [DOD]
- Mortensen K, Christensen LL, Holst JJ, Orskov C. GLP-1 and GIP are colocalized in a subset of endocrine cells in the small intestine. Regul Pept 2003. 114:189-196. [DOD] [CrossRef]
- Parker HE, Reimann F, Gribble FM. Molecular mechanisms underlying nutrient-stimulated incretin secretion. Expert Rev Mol Med 2010. 12:e1. [DOD] [CrossRef]
- Brubaker PL. The glucagon-like peptides: pleiotropic regulators of nutrient homeostasis. Ann N Y Acad Sci 2006. 1070:10-26. [DOD] [CrossRef]
- Meier JJ, Nauck MA. Is the diminished incretin effect in type 2 diabetes just an epi-phenomenon of impaired beta-cell function? Diabetes 2010. 59:1117-1125. [DOD]
- Vilsboll T, Holst JJ. Incretins, insulin secretion and type 2 diabetes mellitus. Diabetologia 2004. 47:357-366. [DOD] [CrossRef]
- Vilsboll T, Krarup T, Deacon CF, Madsbad S, Holst JJ. Reduced postprandial concentrations of intact biologically active glucagon-like peptide 1 in type 2 diabetic patients. Diabetes 2001. 50:609-613. [DOD] [CrossRef]
- Vollmer K, Holst JJ, Baller B, Ellrichmann M, Nauck MA, Schmidt WE, Meier JJ. Predictors of incretin concentrations in subjects with normal, impaired, and diabetic glucose tolerance. Diabetes 2008. 57:678-687. [DOD] [CrossRef]
- Jones IR, Owens DR, Luzio S, Williams S, Hayes TM. The glucose dependent insulinotropic polypeptide response to oral glucose and mixed meals is increased in patients with type 2 (non-insulin-dependent) diabetes mellitus. Diabetologia 1989. 32:668-677. [DOD]
- Vilsboll T, Krarup T, Madsbad S, Holst JJ. Defective amplification of the late phase insulin response to glucose by GIP in obese Type II diabetic patients. Diabetologia 2002. 45:1111-1119. [DOD] [CrossRef]
- Nauck MA, Heimesaat MM, Orskov C, Holst JJ, Ebert R, Creutzfeldt W. Preserved incretin activity of glucagon-like peptide 1 [7-36 amide] but not of synthetic human gastric inhibitory polypeptide in patients with type-2 diabetes mellitus. J Clin Invest 1993. 91:301-307. [DOD] [CrossRef]
- Meier JJ, Hücking K, Holst JJ, Deacon CF, Schmiegel WH, Nauck MA. Reduced insulinotropic effect of gastric inhibitory polypeptide in first-degree relatives of patients with type 2 diabetes. Diabetes 2001. 50:2497-2504. [DOD] [CrossRef]
- Xu G, Kaneto H, Laybutt DR, Duvivier-Kali VF, Trivedi N, Suzuma K, King GL, Weir GC, Bonner-Weir S. Downregulation of GLP-1 and GIP receptor expression by hyperglycemia: possible contribution to impaired incretin effects in diabetes. Diabetes 2007. 56:1551-1558. [DOD] [CrossRef]
- Holst JJ, Gromada J, Nauck MA. The pathogenesis of NIDDM involves a defective expression of the GIP receptor. Diabetologia 1997. 40:984-986. [DOD] [CrossRef]
- Kubota A, Yamada Y, Hayami T, Yasuda K, Someya Y, Ihara Y, Kagimoto S, Watanabe R, Taminato T, Tsuda K, Seino Y. Identification of two missense mutations in the GIP receptor gene: a functional study and association analysis with NIDDM: no evidence of association with Japanese NIDDM subjects. Diabetes 1996. 45:1701-1705. [DOD] [CrossRef]
- Toft-Nielsen MB, Damholt MB, Madsbad S, Hilsted LM, Hughes TE, Michelsen BK, Holst JJ. Determinants of the impaired secretion of glucagon-like peptide-1 in type 2 diabetic patients. J Clin Endocrinol Metab 2001. 86:3717-3723. [DOD] [CrossRef]
- Orskov C, Jeppesen J, Madsbad S, Holst JJ. Proglucagon products in plasma of noninsulin-dependent diabetics and nondiabetic controls in the fasting state and after oral glucose and intravenous arginine. J Clin Invest 1991. 87:415-423. [DOD] [CrossRef]
- Kjems LL, Holst JJ, Volund A, Madsbad S. The influence of GLP-1 on glucose-stimulated insulin secretion: effects on beta-cell sensitivity in type 2 and nondiabetic subjects. Diabetes 2003. 52:380-386. [DOD] [CrossRef]
- Willms B, Werner J, Holst JJ, Orskov C, Creutzfeldt W, Nauck MA. Gastric emptying, glucose responses, and insulin secretion after a liquid test meal: effects of exogenous glucagon-like peptide-1 (GLP-1)-(7-36) amide in type 2 (noninsulin-dependent) diabetic patients. J Clin Endocrinol Metab 1996. 81:327-332. [DOD] [CrossRef]
- Meier JJ, Gallwitz B, Salmen S, Goetze O, Holst JJ, Schmidt WE, Nauck MA. Normalization of glucose concentrations and deceleration of gastric emptying after solid meals during intravenous glucagon-like peptide 1 in patients with type 2 diabetes. J Clin Endocrinol Metab 2003. 88:2719-2725. [DOD] [CrossRef]
- Nauck MA. Incretin-based therapies for type 2 diabetes mellitus: properties, functions, and clinical implications. Am J Med 2011. 124(1 Suppl): S3-S18. [DOD] [CrossRef]
- Meier JJ, Nauck MA. Is the diminished incretin effect in type 2 diabetes just an epi-phenomenon of impaired beta-cell function? Diabetes 2010. 59:1117-1125. [DOD]
- Knop FK, Vilsboll T, Hojberg PV, Larsen S, Madsbad S, Volund A, Holst JJ, Krarup T. Reduced incretin effect in type 2 diabetes: cause or consequence of the diabetic state? Diabetes 2007. 56:1951-1959. [DOD]
- Rask E, Olsson T, Söderberg S, Holst JJ, Tura A, Pacini G, Ahren B. Insulin secretion and incretin hormones after oral glucose in non-obese subjects with impaired glucose tolerance. Metabolism 2004. 53:624-631. [DOD] [CrossRef]
- Ahren B, Larsson H, Holst JJ. Reduced gastric inhibitory polypeptide but normal glucagon-like peptide 1 response to oral glucose in postmenopausal women with impaired glucose tolerance. Eur J Endocrinol 1997. 137:127-131. [DOD] [CrossRef]
- Muscelli E, Mari A, Natali A, Astiarraga BD, Camastra S, Frascerra S, Holst JJ, Ferrannini E. Impact of incretin hormones on beta-cell function in subjects with normal or impaired glucose tolerance. Am J Physiol Endocrinol Metab 2006. 291:E1144-E1150. [DOD] [CrossRef]
- Muscelli E, Mari A, Casolaro A, Camastra S, Seghieri G, Gastaldelli A, Holst JJ, Ferrannini E. Separate impact of obesity and glucose tolerance on the incretin effect in normal subjects and type 2 diabetic patients. Diabetes 2008. 57:1340-1348. [DOD] [CrossRef]
- Theodorakis MJ, Carlson O, Muller DC, Egan JM. Elevated plasma glucose-dependent insulinotropic polypeptide associates with hyperinsulinemia in impaired glucose tolerance. Diabetes Care 2004. 27:1692-1698. [DOD] [CrossRef]
- Kahn SE, Haffner SM, Heise MA, Herman WH, Holman RR, Jones NP, Kravitz BG, Lachin JM, O'Neill MC, Zinman B, Viberti G. Glycemic durability of rosiglitazone, metformin, or glyburide monotherapy. N Engl J Med 2006. 355:2427-2443. [DOD] [CrossRef]
- Nathan DM, Buse JB, Davidson MB, Ferrannini E, Holman RR, Sherwin R, Zinman B. Medical management of hyperglycemia in type 2 diabetes: a consensus algorithm for the initiation and adjustment of therapy: a consensus statement of the American Diabetes Association and the European Association for the Study of Diabetes. Diabetes Care 2009. 32:193-203. [DOD] [CrossRef]
- Schernthaner G, Barnett AH, Betteridge DJ, Carmena R, Ceriello A, Charbonnel B, Hanefeld M, Lehmann R, Malecki MT, Nesto R, et al. Is the ADA/EASD algorithm for the management of type 2 diabetes (January 2009) based on evidence or opinion? A critical analysis. Diabetologia 2010. 53:1258-1269. [DOD] [CrossRef]
- Pratley RE, Gilbert M. Targeting incretins in type 2 diabetes: role of GLP-1 receptor agonists and DPP-4 inhibitors. Rev Diabet Stud 2008. 5(2):73-94. [DOD] [CrossRef]
- Cernea S, Raz I. Therapy in the early stage: incretins. Diabetes Care 2011. 34(Suppl 2):S264-S271. [DOD] [CrossRef]
- Perfetti R, Merkel P. Glucagon-like peptide-1: a major regulator of pancreatic beta-cell function. Eur J Endocrinol 2000. 143:717-725. [DOD] [CrossRef]
- Abraham EJ, Leech CA, Lin JC, Zulewski H, Habener JF. Insulinotropic hormone glucagon-like peptide-1 differentiation of human pancreatic islet-derived progenitor cells into insulin-producing cells. Endocrinology 2002. 143:3152-3161. [DOD] [CrossRef]
- Xu G, Stoffers DA, Habener JF, Bonner-Weir S. Exendin-4 stimulates both beta-cell replication and neogenesis, resulting in increased beta-cell mass and improved glucose tolerance in diabetic rats. Diabetes 1999. 48:2270-2276. [DOD] [CrossRef]
- Mari A, Sallas WM, He YL, Watson C, Ligueros-Saylan M, Dunning BE, Deacon CF, Holst JJ, Foley JE. Vildagliptin, a dipeptidyl peptidase-IV inhibitor, improves model-assessed beta-cell function in patients with type 2 diabetes. J Clin Endocrinol Metabm 2005. 90:4888-4894. [DOD] [CrossRef]
- Fehse F, Trautmann M, Holst JJ, Halseth AE, Nanayakkara N, Nielsen LL, Fineman MS, Kim DD, Nauck MA. Exenatide augments first- and second-phase insulin secretion in response to intravenous glucose in subjects with type 2 diabetes. J Clin Endocrinol Metab 2005. 90:5991-5997. [DOD] [CrossRef]
- Gallwitz B. Preclinical and clinical data on extraglycemic effects of GLP-1 receptor agonists. Rev Diabet Stud 2009. 6(4):247-259. [DOD] [CrossRef]
- Baggio LL, Drucker DJ. Biology of incretins: GLP-1 and GIP. Gastroenterology 2007. 132:2131-2157. [DOD] [CrossRef]
- Nauck MA, Niedereichholz U, Ettler R, Holst JJ, Orskov C, Ritzel R, Schmiegel WH. Glucagon-like peptide 1 inhibition of gastric emptying outweighs its insulinotropic effects in healthy humans. Am J Physiol 1997. 273:E981-E988. [DOD]
- Mudaliar S, Henry RR. Incretin therapies: effects beyond glycemic control. Eur J Intern Med 2009. 20:S319-S328. [DOD] [CrossRef]
- Bose AK, Mocanu MM, Carr RD, Brand CL, Yellon DM. Glucagon-like peptide 1 can directly protect the heart against ischemia/reperfusion injury. Diabetes 2005. 54:146-151. [DOD] [CrossRef]
- Nikolaidis LA, Elahi D, Hentosz T, Doverspike A, Huerbin R, Zourelias L, Stolarski C, Shen YT, Shannon RP. Recombinant glucagon-like peptide-1 increases myocardial glucose uptake and improves left ventricular performance in conscious dogs with pacing-induced dilated cardiomyopathy. Circulation 2004. 110:955-961. [DOD] [CrossRef]
- Nathan DM, Davidson MB, DeFronzo RA, Heine RJ, Henry RR, Pratley R, Zinman B. Impaired fasting glucose and impaired glucose tolerance: implications for care. Diabetes Care 2007. 30:753-759. [DOD] [CrossRef]
- Inzucchi SE, Sherwin RS. The prevention of type 2 diabetes mellitus. Endocrinol Metab Clin North Am 2005. 34:199-219. [DOD] [CrossRef]
- Pan XR, Li GW, Hu YH, Wang JX, Yang WY, An ZX, Hu ZX, Lin J, Xiao JZ, Cao HB, et al. Effects of diet and exercise in preventing NIDDM in people with impaired glucose tolerances. The Da Qing IGT and Diabetes Study. Diabetes Care 1997. 20(4):537-544. [DOD] [CrossRef]
- Diabetes Prevention Program Research Group. Reduction in the incidence of type 2 diabetes with lifestyle intervention or metformin. N Engl J Med 2002. 346:393-403. [DOD] [CrossRef]
- Lindstrom J, Eriksson JG, Valle TT, Aunola S, Cepaitis Z, Hakumäki M, Hämäläinen H, Ilanne-Parikka P, Keinänen-Kiukaanniemi S, Laakso M, et al. Prevention of diabetes mellitus in subjects with impaired glucose tolerance in the Finnish Diabetes Prevention Study: Results from a randomized clinical trial. J Am Soc Nephrol 2003. 14(7 Suppl 2):S108-S113. [DOD] [CrossRef]
- Buchanan TA, Xiang AH, Peters RK, Kjos SL, Marroquin A, Goico J, Ochoa C, Tan S, Berkowitz K, Hodis HN, Azen SP. Preservation of pancreatic β-cell function and prevention of type 2 diabetes by pharmacological treatment of insulin resistance in high risk Hispanic women. Diabetes 2002. 51(9):2796-2803. [DOD] [CrossRef]
- Gerstein HC, Yusuf S, Bosch J, Pogue J, Sheridan P, Dinccag N, Hanefeld M, Hoogwerf B, Laakso M, Mohan V, Shaw J, Zinman B, Holman RR. Effect of rosiglitazone on the frequency of diabetes in patients with impaired glucose tolerance or impaired fasting glucose: A randomised controlled trial. Lancet 2006. 368(9541):1096-1105. [DOD] [CrossRef]
- Bosch J, Yusuf S, Gerstein HC, Pogue J, Sheridan P, Dagenais G, Diaz R, Avezum A, Lanas F, Probstfield J, Fodor G, Holman RR. Effect of ramipril on the incidence of diabetes. N Engl J Med 2006. 355(15):1551-1562. [DOD] [CrossRef]
- Chiasson JL, Josse RG, Gomis R, Hanefeld M, Karasik A, Laakso M. Acarbose treatment and the risk of cardiovascular disease and hypertension in patients with impaired glucose tolerance. The STOP-NIDDM trial. JAMA 2003. 290(4):486-494. [DOD] [CrossRef]
- Pan CY, Gao U, Chen JW, Luo BY, Fu ZZ, Lu JM, Guo XH, Cheng H. Efficacy of acarbose in Chinese subjects with impaired glucose tolerance. Diabetes Res Clin Pract 2003. 61(3):183-190. [DOD] [CrossRef]
- Torgerson JS, Hauptman J, Boldrin MN, Sjöström L. XENical in the prevention of Diabetes in Obese Subjects (XENDOS) study. Diabetes Care 2004. 27:155-161. [DOD] [CrossRef]
- McMurray JJ, Holman RR, Haffner SM, Bethel MA, Holzhauer B, Hua TA, Belenkov Y, Boolell M, Buse JB, Buckley BM, et al. Effect of valsartan on the incidence of diabetes and cardiovascular events. N Engl J Med 2010. 362(16):1477-1490. [DOD] [CrossRef]
- Holman RR, Haffner SM, McMurray JJ, Bethel MA, Holzhauer B, Hua TA, Belenkov Y, Boolell M, Buse JB, Buckley BM, et al. Effect of nateglinide on the incidence of diabetes and cardiovascular events. N Engl J Med 2010. 362(16):1463-1476. [DOD] [CrossRef]
- Rosenstock J, Klaff LJ, Schwartz S, Northrup J, Holcombe JH, Wilhelm K, Trautmann M. Effects of exenatide and lifestyle modification on body weight and glucose tolerance in obese subjects with and without pre-diabetes. Diabetes Care 2010. 33:1173-1175. [DOD] [CrossRef]
- Utzschneider KM, Tong J, Montgomery B, Udayasankar J, Gerchman F, Marcovina SM, Watson CE, Ligueros-Saylan MA, Foley JE, Holst JJ, Deacon CF, Kahn SE. The dipeptidyl peptidase-4 inhibitor vildagliptin improves beta-cell function and insulin sensitivity in subjects with impaired fasting glucose. Diabetes Care 2008. 31:108-113. [DOD] [CrossRef]
- Bock G, Man CD, Micheletto F, Basu R, Rn PD, Laugen J, Deacon CF, Holst JJ, Toffolo G, Cobelli C, Rizza RA, Vella A. The effect of DPP-4 inhibition with sitagliptin on incretin secretion and on fasting and postprandial glucose turnover in subjects with impaired fasting glucose. Clin Endocrinol (Oxf) 2010. 73:189-196. [DOD]
- Rosenstock J, Foley JE, Rendell M, Landin-Olsson M, Holst JJ, Deacon CF, Rochotte E, Baron MA. Effects of the dipeptidyl peptidase-IV inhibitor vildagliptin on incretin hormones, islet function, and postprandial glycemia in subjects with impaired glucose tolerance. Diabetes Care 2008. 31:30-35. [DOD] [CrossRef]
- Handelsman Y, Mechanick JI, Blonde L, Grunberger G, Bloomgarden ZT, Bray GA, Dagogo-Jack S, Davidson JA, Einhorn D, Ganda O, et al. American Association of Clinical Endocrinologists Medical Guidelines for Clinical Practice for developing a diabetes mellitus comprehensive care plan. Endocr Pract 2011. 17(Suppl 2):1-53. [DOD]
- The National Collaborating Centre for Chronic Conditions. Type 2 diabetes. National Clinical Guideline for Management in Primary and Secondary Care (update). Royal College of Physicians, London, 2008. [DOD]
- Bhattacharyya OK, Estey EA, Cheng AY. Update on the Canadian Diabetes Association 2008 clinical practice guidelines. Can Fam Physician 2009. 55:39-43. [DOD]
- Migoya EM, Bergeron R, Miller JL, Snyder RN, Tanen M, Hilliard D, Weiss B, Larson P, Gutierrez M, Jiang G, et al. Dipeptidyl peptidase-4 inhibitors administered in combination with metformin result in an additive increase in the plasma concentration of active GLP-1. Clin Pharmacol Ther 2010. 88:801-808. [DOD] [CrossRef]
- Maida A, Lamont BJ, Cao X, Drucker DJ. Metformin regulates the incretin receptor axis via a pathway dependent on peroxisome proliferator-activated receptor-alpha in mice. Diabetologia 2011. 54:339-349. [DOD] [CrossRef]
- Eldor R, Raz I. The individualized target HbA1c: a new method for improving macrovascular risk and glycemia without hypoglycemia and weight gain. Rev Diabet Stud 2009. 6(1):6-12. [DOD] [CrossRef]
- Amori RE, Lau J, Pittas AG. Efficacy and safety of incretin therapy in type 2 diabetes: systematic review and meta-analysis. JAMA 2007. 298:194-206. [DOD] [CrossRef]
- Fakhoury WK, Lereun C, Wright D. A meta-analysis of placebo-controlled clinical trials assessing the efficacy and safety of incretin-based medications in patients with type 2 diabetes. Pharmacology 2010. 86:44-57. [DOD] [CrossRef]
- Moretto TJ, Milton DR, Ridge TD, Macconell LA, Okerson T, Wolka AM, Brodows RG. Efficacy and tolerability of exenatide monotherapy over 24 weeks in antidiabetic drug-naive patients with type 2 diabetes: a randomized, double-blind, placebo-controlled, parallel-group study. Clin Ther 2008. 30:1448-1460. [DOD] [CrossRef]
- Poon T, Nelson P, Shen L, Mihm M, Taylor K, Fineman M, Kim D. Exenatide improves glycemic control and reduces body weight in subjects with type 2 diabetes: a dose-ranging study. Diabetes Technol Ther 2005. 7:467-477. [DOD] [CrossRef]
- Seino Y, Rasmussen MF, Zdravkovic M, Kaku K. Dose-dependent improvement in glycemia with once-daily liraglutide without hypoglycemia or weight gain: A double-blind, randomized, controlled trial in Japanese patients with type 2 diabetes. Diabetes Res Clin Pract 2008. 81:161-168. [DOD] [CrossRef]
- Vilsboll T, Zdravkovic M, Le-Thi T, Krarup T, Schmitz O, Courreges JP, Verhoeven R, Buganova I, Madsbad S. Liraglutide, a long-acting human glucagon-like peptide-1 analog, given as monotherapy significantly improves glycemic control and lowers body weight without risk of hypoglycemia in patients with type 2 diabetes. Diabetes Care 2007. 30:1608-1610. [DOD] [CrossRef]
- Garber A, Henry R, Ratner R, Garcia-Hernandez PA, Rodriguez-Pattzi H, Olvera-Alvarez I, Hale PM, Zdravkovic M, Bode B. Liraglutide versus glimepiride monotherapy for type 2 diabetes (LEAD-3 Mono): a randomised, 52-week, phase III, double-blind, parallel-treatment trial. Lancet 2009. 373:473-481. [DOD] [CrossRef]
- Aschner P, Kipnes MS, Lunceford JK, Sanchez M, Mickel C, Williams-Herman DE, Sitagliptin Study 021 Group. Effect of the dipeptidyl peptidase-4 inhibitor sitagliptin as monotherapy on glycemic control in patients with type 2 diabetes. Diabetes Care 2006. 29:2632-2637. [DOD] [CrossRef]
- Hanefeld M, Herman GA, Wu M, Mickel C, Sanchez M, Stein PP. Sitagliptin Study 014 Investigators. Once-daily sitagliptin, a dipeptidyl peptidase-4 inhibitor, for the treatment of patients with type 2 diabetes. Curr Med Res Opin 2007. 23:1329-1339. [DOD] [CrossRef]
- Mohan V, Yang W, Son HY, Xu L, Noble L, Langdon RB, Amatruda JM, Stein PP, Kaufman KD. Efficacy and safety of sitagliptin in the treatment of patients with type 2 diabetes in China, India, and Korea. Diabetes Res Clin Pract 2009. 83:106-116. [DOD] [CrossRef]
- Nonaka K, Kakikawa T, Sato A, Okuyama K, Fujimoto G, Kato N, Suzuki H, Hirayama Y, Ahmed T, Davies MJ, Stein PP. Efficacy and safety of sitagliptin monotherapy in Japanese patients with type 2 diabetes. Diabetes Res Clin Pract 2008. 79:291-298. [DOD] [CrossRef]
- Scott R, Wu M, Sanchez M, Stein P. Efficacy and tolerability of the dipeptidyl peptidase-4 inhibitor sitagliptin as monotherapy over 12 weeks in patients with type 2 diabetes. Int J Clin Pract 2007. 61:171-180. [DOD] [CrossRef]
- Raz I, Hanefeld M, Xu L, Caria C, Williams-Herman D, Khatami H. Sitagliptin Study 023 Group. Efficacy and safety of the dipeptidyl peptidase-4 inhibitor sitagliptin as monotherapy in patients with type 2 diabetes mellitus. Diabetologia 2006. 49:2564-2571. [DOD] [CrossRef]
- Ristic S, Byiers S, Foley J, Holmes D. Improved glycaemic control with dipeptidyl peptidase-4 inhibition in patients with type 2 diabetes: vildagliptin (LAF237) dose response. Diabetes Obes Metab 2005. 7:692-698. [DOD] [CrossRef]
- Dejager S, Razac S, Foley JE, Schweizer A. Vildagliptin in drug-naïve patients with type 2 diabetes: a 24-week, double-blind, randomized, placebo-controlled, multiple-dose study. Horm Metab Res 2007. 39:218-223. [DOD] [CrossRef]
- Kikuchi M, Abe N, Kato M, Terao S, Mimori N, Tachibana H. Vildagliptin dose-dependently improves glycemic control in Japanese patients with type 2 diabetes mellitus. Diabetes Res Clin Pract 2009. 83:233-240. [DOD] [CrossRef]
- Pi-Sunyer FX, Schweizer A, Mills D, Dejager S. Efficacy and tolerability of vildagliptin monotherapy in drug-naïve patients with type 2 diabetes. Diabetes Res Clin Pract 2007. 76:132-138. [DOD] [CrossRef]
- Scherbaum WA, Schweizer A, Mari A, Nilsson PM, Lalanne G, Wang Y, Dunning BE, Foley JE. Evidence that vildagliptin attenuates deterioration of glycaemic control during 2-year treatment of patients with type 2 diabetes and mild hyperglycaemia. Diabetes Obes Metab 2008. 10:1114-1124. [DOD] [CrossRef]
- Rosenstock J, Aguilar-Salinas C, Klein E, Nepal S, List J, Chen R. Effect of saxagliptin monotherapy in treatment-naïve patients with type 2 diabetes. Curr Med Res Opin 2009. 25:2401-2411. [DOD] [CrossRef]
- Rosenstock J, Sankoh S, List JF. Glucose-lowering activity of the dipeptidyl peptidase-4 inhibitor saxagliptin in drug-naive patients with type 2 diabetes. Diabetes Obes Metab 2008. 10:376-386. [DOD] [CrossRef]
- Schweizer A, Couturier A, Foley JE, Dejager S. Comparison between vildagliptin and metformin to sustain reductions in HbA(1c) over 1 year in drug-naïve patients with type 2 diabetes. Diabet Med 2007. 24:955-961. [DOD] [CrossRef]
- Del Prato S, Bianchi C, Marchetti P. Beta-cell function and anti-diabetic pharmacotherapy. Diabetes Metab Res Rev 2007. 23:518-527. [DOD] [CrossRef]
- Nauck M, Smith U. Incretin-based therapy: how do incretin mimetics and DPP-4 inhibitors fit into treatment algorithms for type 2 diabetic patients? Best Pract Res Clin Endocrinol Metab 2009. 23:513-523. [DOD]
- Wajcberg E, Tavaria A. Exenatide: clinical aspects of the first incretin-mimetic for the treatment of type 2 diabetes mellitus. Expert Opin Pharmacother 2009. 10:135-142. [DOD] [CrossRef]
- DeFronzo RA, Ratner RE, Han J, Kim DD, Fineman MS, Baron AD. Effects of exenatide (exendin-4) on glycemic control and weight over 30 weeks in metformin-treated patients with type 2 diabetes. Diabetes Care 2005. 28:1092-1100. [DOD] [CrossRef]
- Nauck M, Frid A, Hermansen K, Shah NS, Tankova T, Mitha IH, Zdravkovic M, Düring M, Matthews DR. Efficacy and safety comparison of liraglutide, glimepiride, and placebo, all in combination with metformin, in type 2 diabetes: the LEAD (liraglutide effect and action in diabetes)-2 study. Diabetes Care 2009. 32:84-90. [DOD] [CrossRef]
- Buse JB, Henry RR, Han J, Kim DD, Fineman MS, Baron AD, Exenatide-113 Clinical Study Group. Effects of exenatide (exendin-4) on glycemic control over 30 weeks in sulfonylurea-treated patients with type 2 diabetes. Diabetes Care 2004. 27:2628-2635. [DOD] [CrossRef]
- Marre M, Shaw J, Brändle M, Bebakar WM, Kamaruddin NA, Strand J, Zdravkovic M, Le Thi TD, Colagiuri S. Liraglutide, a once-daily human GLP-1 analogue, added to a sulphonylurea over 26 weeks produces greater improvements in glycaemic and weight control compared with adding rosiglitazone or placebo in subjects with Type 2 diabetes (LEAD-1 SU). Diabet Med 2009. 26:268-278. [DOD] [CrossRef]
- Zinman B, Hoogwerf BJ, Duran Garcia S, Milton DR, Giaconia JM, Kim DD, Trautmann ME, Brodows RG. The effect of adding exenatide to a thiazolidinedione in suboptimally controlled type 2 diabetes: a randomized trial. Ann Intern Med 2007. 146:477-485. [DOD]
- Scott R, Loeys T, Davies MJ, Engel SS. Efficacy and safety of sitagliptin when added to ongoing metformin therapy in patients with type 2 diabetes. Diabetes Obes Metab 2008. 10:959-969. [DOD] [CrossRef]
- Charbonnel B, Karasik A, Liu J, Wu M, Meininger G. Efficacy and safety of the dipeptidyl peptidase-4 inhibitor sitagliptin added to ongoing metformin therapy in patients with type 2 diabetes inadequately controlled with metformin alone. Diabetes Care 2006. 29:2638-2643. [DOD] [CrossRef]
- Goldstein BJ, Feinglos MN, Lunceford JK, Johnson J, Williams-Herman DE. Effect of initial combination therapy with sitagliptin, a dipeptidyl peptidase-4 inhibitor, and metformin on glycemic control in patients with type 2 diabetes. Diabetes Care 2007. 30:1979-1987. [DOD] [CrossRef]
- Raz I, Chen Y, Wu M, Hussain S, Kaufman KD, Amatruda JM, Langdon RB, Stein PP, Alba M. Efficacy and safety of sitagliptin added to ongoing metformin therapy in patients with type 2 diabetes. Curr Med Res Opin 2008. 24:537-550. [DOD] [CrossRef]
- Ahren B, Gomis R, Standl E, Mills D, Schweizer A. Twelve- and 52-week efficacy of the dipeptidyl peptidase IV inhibitor LAF237 in metformin-treated patients with type 2 diabetes. Diabetes Care 2004. 27:2874-2880. [DOD] [CrossRef]
- Bosi E, Camisasca RP, Collober C, Rochotte E, Garber AJ. Effects of vildagliptin on glucose control over 24 weeks in patients with type 2 diabetes inadequately controlled with metformin. Diabetes Care 2007. 30:890-895. [DOD] [CrossRef]
- Goodman M, Thurston H, Penman J. Efficacy and tolerability of vildagliptin in patients with type 2 diabetes inadequately controlled with metformin monotherapy. Horm Metab Res 2009. 41:368-373. [DOD] [CrossRef]
- Ferrannini E, Fonseca V, Zinman B, Matthews D, Ahren B, Byiers S, Shao Q, Dejager S. Fifty-two-week efficacy and safety of vildagliptin vs. glimepiride in patients with type 2 diabetes mellitus inadequately controlled on metformin monotherapy. Diabetes Obes Metab 2009. 11:157-166. [DOD] [CrossRef]
- Jadzinsky M, Pfützner A, Paz-Pacheco E, Xu Z, Allen E, Chen R. Saxagliptin given in combination with metformin as initial therapy improves glycaemic control in patients with type 2 diabetes compared with either monotherapy: a randomized controlled trial. Diabetes Obes Metab 2009. 11:611-622. [DOD] [CrossRef]
- DeFronzo RA, Hissa MN, Garber AJ, Luiz Gross J, Yuyan Duan R, Ravichandran S, Chen RS. The efficacy and safety of saxagliptin when added to metformin therapy in patients with inadequately controlled type 2 diabetes with metformin alone. Diabetes Care 2009. 32:1649-1655. [DOD] [CrossRef]
- Hermansen K, Kipnes M, Luo E, Fanurik D, Khatami H, Stein P. Efficacy and safety of the dipeptidyl peptidase-4 inhibitor, sitagliptin, in patients with type 2 diabetes mellitus inadequately controlled on glimepiride alone or on glimepiride and metformin. Diabetes Obes Metab 2007. 9:733-745. [DOD] [CrossRef]
- Garber AJ, Foley JE, Banerji MA, Ebeling P, Gudbjörnsdottir S, Camisasca RP, Couturier A, Baron MA. Effects of vildagliptin on glucose control in patients with type 2 diabetes inadequately controlled with a sulphonylurea. Diabetes Obes Metab 2008. 10:1047-1056. [DOD] [CrossRef]
- Chacra AR, Tan GH, Apanovitch A, Ravichandran S, List J, Chen R. Saxagliptin added to a submaximal dose of sulphonylurea improves glycaemic control compared with uptitration of sulphonylurea in patients with type 2 diabetes: a randomised controlled trial. Int J Clin Pract 2009. 63:1395-1406. [DOD] [CrossRef]
- Rosenstock J, Brazg R, Andryuk PJ, Lu K, Stein P. Efficacy and safety of the dipeptidyl peptidase-4 inhibitor sitagliptin added to ongoing pioglitazone therapy in patients with type 2 diabetes: a 24-week, multicenter, randomized, double-blind, placebo-controlled, parallel-group study. Clin Ther 2006. 28:1556-1568. [DOD] [CrossRef]
- Garber AJ, Schweizer A, Baron MA, Rochotte E, Dejager S. Vildagliptin in combination with pioglitazone improves glycaemic control in patients with type 2 diabetes failing thiazolidinedione monotherapy: a randomized, placebo-controlled study. Diabetes Obes Metab 2007. 9:166-174. [DOD] [CrossRef]
- Hollander P, Li J, Allen E, Chen R. Saxagliptin added to a thiazolidinedione improves glycemic control in patients with type 2 diabetes and inadequate control on thiazolidinedione alone. J Clin Endocrinol Metab 2009. 94:4810-4819. [DOD] [CrossRef]
- Hollander PL, Jia Li, Frederich R, Allen E, Chen R. Safety and efficacy of saxagliptin added to thiazolidinedione over 76 weeks in patients with type 2 diabetes mellitus. Diab Vasc Dis Res 2011. 8:125-135. [DOD] [CrossRef]
- Fonseca V, Schweizer A, Albrecht D, Baron MA, Chang I, Dejager S. Addition of vildagliptin to insulin improves glycaemic control in type 2 diabetes. Diabetologia 2007. 50:1148-1155. [DOD] [CrossRef]
- Ling Y, Kaufman KD, Amatruda JM, Engel SS, Katz L. Efficacy and safety of sitagliptin when added to insulin therapy in patients with type 2 diabetes. Diabetes Obes Metab 2010. 12:167-177. [DOD] [CrossRef]
- Kendall DM, Riddle MC, Rosenstock J, Zhuang D, Kim DD, Fineman MS, Baron AD. Effects of exenatide (exendin-4) on glycemic control over 30 weeks in patients with type 2 diabetes treated with metformin and a sulfonylurea. Diabetes Care 2005. 28:1083-1091. [DOD] [CrossRef]
- Nauck MA, Duran S, Kim D, Johns D, Northrup J, Festa A, Brodows R, Trautmann M. A comparison of twice-daily exenatide and biphasic insulin aspart in patients with type 2 diabetes who were suboptimally controlled with sulfonylurea and metformin: a non-inferiority study. Diabetologia 2007. 50:259-267. [DOD] [CrossRef]
- Heine RJ, Van Gaal LF, Johns D, Mihm MJ, Widel MH, Brodows RG. Exenatide versus insulin glargine in patients with suboptimally controlled type 2 diabetes: a randomized trial. Ann Intern Med 2005. 143:559-569. [DOD]
- Russell-Jones D, Vaag A, Schmitz O, Sethi BK, Lalic N, Antic S, Zdravkovic M, Ravn GM, Simo R. Liraglutide vs insulin glargine and placebo in combination with metformin and sulfonylurea therapy in type 2 diabetes mellitus (LEAD-5 met+SU): a randomised controlled trial. Diabetologia 2009. 52:2046-2055. [DOD] [CrossRef]
- Zinman B, Gerich J, Buse JB, Lewin A, Schwartz S, Raskin P, Hale PM, Zdravkovic M, Blonde L. Efficacy and safety of the human glucagon-like peptide-1 analog liraglutide in combination with metformin and thiazolidinedione in patients with type 2 diabetes (LEAD-4 Met+TZD). Diabetes Care 2009. 32:1224-1230. [DOD] [CrossRef]
- Gallwitz B, Böhmer M, Segiet T, Mölle A, Milek K, Becker B, Helsberg K, Petto H, Peters N, Bachmann O. Exenatide twice daily versus premixed insulin aspart 70/30 in metformin-treated patients with type 2 diabetes: a randomized 26-week study on glycemic control and hypoglycemia. Diabetes Care 2011. 34:604-606. [DOD] [CrossRef]
- Davies MJ, Donnelly R, Barnett AH, Jones S, Nicolay C, Kilcoyne A. Exenatide compared with long-acting insulin to achieve glycaemic control with minimal weight gain in patients with type 2 diabetes: results of the Helping Evaluate Exenatide in patients with diabetes compared with Long-Acting insulin (HEELA) study. Diabetes Obes Metab 2009. 11:1153-1162. [DOD] [CrossRef]
- Barnett AH, Burger J, Johns D, Brodows R, Kendall DM, Roberts A, Trautmann ME. Tolerability and efficacy of exenatide and titrated insulin glargine in adult patients with type 2 diabetes previously uncontrolled with metformin or a sulfonylurea: a multinational, randomized, open-label, two-period, crossover noninferiority trial. Clin Ther 2007. 29:2333-2348. [DOD] [CrossRef]
- Pratley RE, Nauck M, Bailey T, Montanya E, Cuddihy R, Filetti S, Thomsen AB, Sondergaard RE, Davies M. Liraglutide versus sitagliptin for patients with type 2 diabetes who did not have adequate glycaemic control with metformin: a 26-week, randomised, parallel-group, open-label trial. Lancet 2010. 375:1447-1456. [DOD] [CrossRef]
- Bergenstal RM, Wysham C, Macconell L, Malloy J, Walsh B, Yan P, Wilhelm K, Malone J, Porter LE. Efficacy and safety of exenatide once weekly versus sitagliptin or pioglitazone as an adjunct to metformin for treatment of type 2 diabetes (DURATION-2): a randomised trial. Lancet 2010. 376:431-439. [DOD] [CrossRef]
- DeFronzo RA, Okerson T, Viswanathan P, Guan X, Holcombe JH, MacConell L. Effects of exenatide versus sitagliptin on postprandial glucose, insulin and glucagon secretion, gastric emptying, and caloric intake: a randomized, cross-over study. Curr Med Res Opin 2008. 24:2943-2952. [DOD] [CrossRef]
- Berg JK, Shenouda SK, Heilmann CR, Gray AL, Holcombe JH. Effects of exenatide twice daily versus sitagliptin on 24-hour glucose, glucoregulatory, and hormonal measures: a randomized, double-blind, crossover study. Diabetes Obes Metab 2011. In press. [DOD]
- Verspohl EJ. Novel therapeutics for type 2 diabetes: incretin hormone mimetics (glucagon-like peptide-1 receptor agonists) and dipeptidyl peptidase-4 inhibitors. Pharmacol Ther 2009. 124:113-138. [DOD] [CrossRef]
- Drucker DJ, Nauck MA. The incretin system: glucagon-like peptide-1 receptor agonists and dipeptidyl peptidase-4 inhibitors in type 2 diabetes. Lancet 2006. 368:1696-1705. [DOD] [CrossRef]
- Ahren B. DPP-4 inhibitors. Best Pract Res Clin Endocrinol Metab 2007. 21:517-533. [DOD] [CrossRef]
This article has been cited by other articles:
|
Correlation Between Baseline Characteristics and Clinical Outcomes in a Large Population of Diabetes Patients Treated with Liraglutide in a Real-World Setting in Italy
Lapolla A, Frison V, Bettio M, Pos MD, Rocchini P, Panebianco G, Tadiotto F, Tos VD, D'Ambrosio M, Marangoni A, et al.
Clin Ther 2015. pii:S0149-2918(14)00829-7
|
|
|
Differential acute postprandial effects of processed meat and isocaloric vegan meals on the gastrointestinal hormone response in subjects suffering from type 2 diabetes and healthy controls: a randomized crossover study
Belinova L, Kahleova H, Malinska H, Topolcan O, Vrzalova J, Oliyarnyk O, Kazdova L, Hill M, Pelikanova T
Plos One 2014. 9(9):e107561
|
|
|
Managing recent-onset diabetes: choosing durable, well-tolerated therapies and understanding the role of incretin-based therapies
Shubrook JH Jr
J Am Osteopath Assoc 2014. 114(5 Suppl 2):S6-S13
|
|
|
Benefits of healthy adipose tissue in the treatment of diabetes
Gunawardana SC
World J Diabetes 2014. 5(4):420-430
|
|
|
Effects of green tea extract on insulin resistance and glucagon-like peptide 1 in patients with type 2 diabetes and lipid abnormalities: a randomized, double-blinded, and placebo-controlled trial.
Liu CY, Huang CJ, Huang LH, Chen IJ, Chiu JP, Hsu CH
Plos One 2014. 9(3):e91163
|
|
|
Effect of combined therapy of human Wharton's jelly-derived mesenchymal stem cells from umbilical cord with sitagliptin in type 2 diabetic rats
Hu J, Wang F, Sun R, Wang Z, Yu X, Wang L, Gao H, Zhao W, Yan S, Wang Y
Endocrine 2014. 45(2):279-287
|
|
|
Diabetes and beta cell function: from mechanisms to evaluation and clinical implications
Cernea S, Dobreanu M
Biochem Med (Zagreb) 2013. 23(3):266-280
|
|
|
Adipose tissue, hormones, and treatment of type 1 diabetes
Gunawardana SC
Curr Diabetes Rep 2012. 12(5):542-550
|
|
|
The experience with the clinical application of liralgutide (victosa), the first analog of human glucagon-like peptide-1 in the patients with type 2 diabetes mellitus - expanding the range of possibilities
Ostroukhova EN, Khmel'nitskii OK, Krasil'nikova EI, Davidenko KS
Probl Endocrinol 2012. 58(3):51-55
|
|
|
The continuing need for drug development and clinical trials in type 2 diabetes and its complications: introduction to the RDS Special Issue
Raz I, Gallwitz B
Rev Diabet Stud 2011. 8(3):288-292
|
|
|